The focus of colostrum feeding regimens is generally centred around providing an adequate amount of immunoglobulins to the neonatal calf within the first 24 hours of life. On this basis, measurement of its quality is usually based upon immunoglobulin concentration, and provision of 150–200 g IgG via 3–4 litres of colostrum containing at least 50 g/litre IgG has been recommended (Godden et al, 2019). High quality colostrum should also have minimal bacterial contamination and many commercial technologies for hygienic collection and storage exist (Figure 1). Samples collected for bacterial culture should have a total bacterial count and a total coliform count of <100 000 cfu/ml and <10 000 cfu/ml respectively (McGuirk and Collins 2004; Phipps et al, 2016). However, the quality of colostrum is not determined by these factors alone.
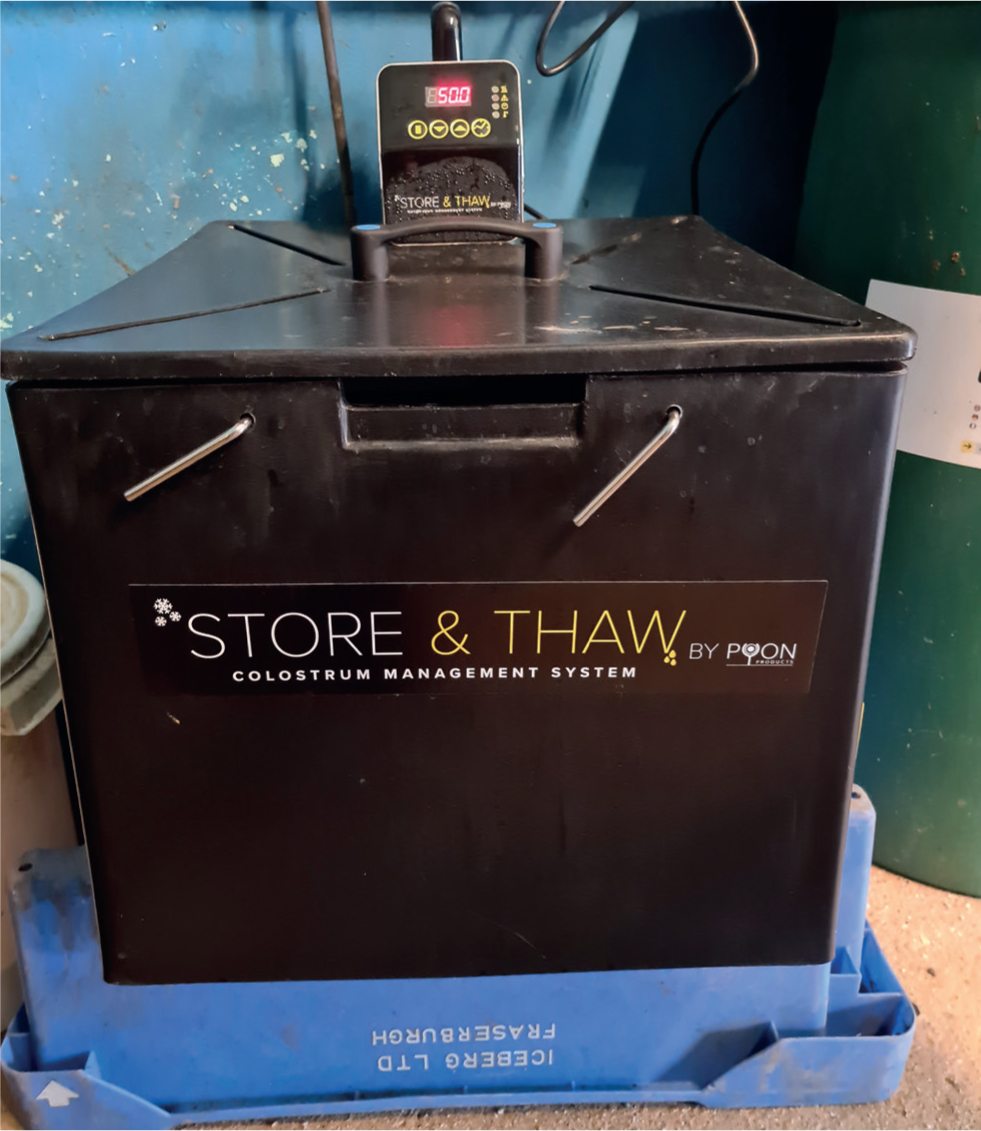
Other constituents within colostrum, commonly referred to as ‘non-nutritive’ components, play a role in supporting the neonate, either because of their nutritional value or the biological activity that they exhibit (Elfstrand et al, 2002). For example, colostrum provides carbohydrates, lipids, proteins, minerals and vitamins, as well as hormones, cytokines, growth factors, lactoferrin, oligosaccharides, somatic cells and microRNAs (Blum and Hammon, 2000). This article provides readers with an understanding of the components of bovine colostrum from a nutritive and a non-nutritive perspective, outlining their functions and the effect of colostrum management practices on their preservation.
Colostrogenesis
The process of colostrogenesis can be defined as the secretion of colostrum components from polarised epithelial cells located within the alveoli of the mammary gland (Cristea and Polyak, 2018; Baumrucker et al, 2021). It begins around 3–4 weeks before calving, under the influence of prolactin and other lactogenic hormones, with peak production occurring 1–3 days before parturition and ceasing shortly after calving (Godden, 2008).
Bovine colostrum consists of a combination of lacteal secretions and constituents of blood serum, particularly immunoglobulins (Foley and Otterby, 1978). Neonatal immunoglobulin receptors, expressed by mammary alveolar epithelial cells, facilitate the transfer of IgG1 (and, to a lesser extent, IgG2) from serum into colostrum via a process of transcytosis, whereas IgA and IgM are synthesised by plasmacytes within the mammary gland (Larson et al, 1980; Tizard, 2018). IgG1 is the predominant immunoglobulin present within bovine colostrum, accounting for around 70–80% of the total content. IgG2, IgA and IgM are present at much lower concentrations (Godden et al, 2019; Puppel et al, 2019). Following parturition in the dam, carrier-mediated transport of IgG1 into the udder ceases, passive resorption into the blood begins, and dilution of IgG1 by lower concentration transition milk occurs (Moore et al, 2005). It is because of these processes that colostrum should be collected as promptly as possible following calving, to ensure harvested IgG content is as high as possible. Nonetheless, even following timely collection, the concentration of immunoglobulin isotypes within colostrum at the time of harvest is highly variable, affected by factors including parity, colostrum yield, breed, genetics and dry period length (Ahmann et al, 2021). On this basis, producers should be encouraged to measure the quality of colostrum at collection. If it is kept, treated or preserved in any way, it should be tested again before feeding. For further information, readers are directed to an article by Glover (2021) which provides practical guidelines for the measurement of colostrum quality.
Nutritive components of colostrum
In comparison to whole milk, the total solids concentration of first milking colostrum is higher and is associated with a higher protein and fat content (Godden, 2008). Lipids (and to a lesser extent, lactose) represent a crucial energy source for the neonatal calf, which has a limited supply of energy reserves in the form of body fat at birth (Morrill et al, 2012). Carbohydrates, including oligosaccharides and lactose, glycolipids, glycopeptides and glycoproteins, as well as small amounts of other sugars such as glucose, fructose, glucosamine and galactosamine, are also present (Gopal and Gill, 2000). The concentration of lactose is low in colostrum, increasing in an inverse relationship with fat with each milking as the transition to whole milk occurs, until it becomes the predominant energy source to the calf (McGrath et al, 2016).
Nutritive proteins within bovine colostrum include caseins and whey proteins, the latter including β-lactoglobulin and α-lactalbumin (Baumrucker et al, 2021). Albumin and amino acids are also present. Caseins are insoluble proteins defined based upon their amino acid composition, whereas whey proteins may be more simply defined as milk proteins which remain soluble after the precipitation of caseins (Farrell et al, 2004). Importantly, the absorption of immunoglobulins during the first 24 hours of life is considered non-specific, raising questions about the effects of differences in nutritive protein concentration within colostrum on immunoglobulin absorption (Lopez and Heinrichs, 2022). As such, it has been demonstrated that colostrum replacers or supplements containing a high concentration of casein will affect serum total protein concentration, but not serum IgG concentrations, when measured directly. It is on this basis that alternative thresholds for defining failure of passive transfer measured indirectly using refractometry have been suggested in herds where colostrum replacers or supplements are fed (Davenport et al, 2000; Glover, 2021). For example, in a study comparing serum total protein with immunoglobulin G concentration in over 1250 calves fed colostrum replacer, the total protein cut-off with the optimum balance of sensitivity and specificity for prediction of an IgG concentration below 10 g/litre was 4.9 g/dl (Lopez et al, 2021).
Bovine colostrum also contains higher concentrations of fatsoluble and water-soluble vitamins, many of which do not cross the placenta in significant quantities (Godden et al, 2019). Vitamin A in the forms of retinol, retinoic acid, retinyl esters and carotenoids, vitamin E in the forms of tocopherols and tocotrienols, as well as vitamins K, D and C are all present in greater concentrations than in whole milk (McGrath et al, 2016; Arslan et al, 2021). The concentration of a number of minerals is also higher, notably calcium, magnesium, phosphate and sodium (Foley and Otterby, 1978). The high lactational demand for calcium within colostrum (and milk) is an important contributory factor to (sub)clinical hypocalcaemia in adult cattle. Production of 10 kg of bovine colostrum requires about 20–40 g of calcium, 10 times that of the circulating plasma pool and such is the demand that a transient period of hypocalcaemia may be physiologically normal in high-yielding cows (McArt and Neves, 2020; Wilkens et al, 2020).
Non-nutritive components of colostrum
Although they may be classified in various ways, non-nutritive components of bovine colostrum can generally be considered as immunoglobulins, microRNAs, bioactive molecules and somatic cells (Hassiotou and Hartmann, 2014; Bartol et al, 2017).
Immunoglobulins
Immunoglobulins are the main non-nutritive protein within bovine colostrum, responsible for its high protein content which is about 4.5 times that of whole milk (Lopez and Heinrichs, 2022). IgG1 is the predominant immunoglobulin present in colostrum, found highly concentrated at levels 5–10 times that of serum. At the peak of colostrogenesis up to 500 g/week of IgG may be transferred to mammary secretions (Barrington et al, 2001). Immunoglobulin is an essential part of colostrum, since the cotyledonary synepitheliochorial structure of the bovine placenta ensures that fetal and maternal blood supplies remain separate during gestation, inhibiting the transfer of immunoglobulins from dam to calf in utero (Peter, 2013). As a consequence, the calf is born agammaglobulinaemic and is reliant upon the enteric absorption of immunoglobulins from colostrum via non-selective pinocytosis during the first 24 hours after birth, as a means of providing maternally derived antibodies (Geiger, 2020).
This process is described by the term ‘passive transfer’, which is crucial for providing a degree of antibody-mediated immunity. The concentration of antigen-specific antibodies will be influenced by dam exposure, either naturally or as a consequence of prepartum vaccination (Lefkaditis et al, 2020; Maier et al, 2022). The ability of the enterocytes to absorb immunoglobulins decreases rapidly soon after birth, resulting in ‘closure’ of the intestines by 24–36 hours postpartum (Smith et al, 2021).
The extent of passive transfer can be measured directly or indirectly, with a general consensus that a serum IgG concentration of <10 mg/ml can be defined as ‘failure’ of passive transfer (Glover, 2021; Robbers et al, 2021). In practice, measurement of total serum solids concentration is common, with thresholds between 5.0 g/dl and 5.5 g/dl generally used depending on the desired sensitivity and specificity of the test (Cuttance et al, 2017). From a practical standpoint, higher serum IgG concentrations are associated with a reduced risk of morbidity and producers should be striving to not only minimise ‘failure’ of passive transfer, but also to far exceed this threshold (Urie et al, 2018). On this basis, an expert consensus in North America has proposed using revised targets whereby passive transfer measurements are classified into four categories: ‘excellent, good, fair and poor’, based upon serum IgG measurements of ≥25.0, 18.0–24.9, 10.0–17.9 and <10 g/litre respectively (Table 1) (Lombard et al, 2020). Nonetheless, when considered from an epidemiological standpoint, passive transfer represents a single contributing factor to ‘calf susceptibility’, whereby the aetiology of clinical disease will also be determined by pathogen and environmental factors (Thrusfield and Christley, 2018). On this basis, good passive transfer may not be sufficient to prevent morbidity or mortality in circumstances where pathogen challenge is high or nutrition or environmental conditions are suboptimal (Weaver et al, 2000; Johnson et al, 2021).
Table 1. Revised classification system for measurement of passive transfer status in neonatal calves and proposed herd-level targets, based upon expert consensus in North America
Category | Serum IgG (g/litre) | Serum total protein (g/dl) | Target proportion of calves within the category |
---|---|---|---|
Excellent | ≥25 | ≥6.2 | >40% |
Good | 18.0–24.9 | 5.8–6.1 | ≈30% |
Fair | 10.0–17.9 | 5.1–5.7 | ≈20% |
Poor | <10 | <5.1 | <10 |
For further information regarding failure of passive transfer and its diagnosis, readers are directed to Glover (2021).
MicroRNAs
These are small, non-coding, single-stranded RNA molecules. They are associated with two main functions, contributing locally to mammary development in the dam, while also providing oral biochemical signalling as a means of communication between dam and calf, via regulation of gene expression (van Hese et al, 2020). Encased in exosomes which protect them from the acidic environment of the abomasum, colostrum-borne microRNAs within the intestines may act locally, or be absorbed from the intestine into the systemic circulation (Bartol et al, 2017). In bovine species they have been implicated in the development of the intestinal epithelium, as well as the development and regulation of the bovine immune system (van Hese et al, 2020).
Bioactive factors
Maternally derived bioactive factors within colostrum include growth factors, peptide and steroid hormones (such as ghrelin, leptin, adiponectin and glucocorticoids), cytokines, enzymes, oligosaccharides and peptides such as lactoferrin (Park and Nam, 2015). They exert local effects on development of the gastrointestinal tract and microbiota, as well as systemic effects including the activation of metabolic and endocrine systems such as the somatotrophic axis.
Factors transmitted within mammary secretions can act as developmental signals, exerting postnatal effects on the adult pheno-type (Bartol et al, 2017). This mode of signalling has been coined the ‘lactocrine hypothesis’. Perhaps the most notable effect of colostrum deficiency has been described in the pig. Deprivation of relaxin, found at its highest concentration within sows' milk during the first 48 hours after birth, was associated with alterations in uterine development and endometrial gene expression, with a significant reduction in fecundity (Bagnell and Bartol, 2019). Extrapolation of the long-term epigenetic effects of colostrum feeding in calves specifically is less well researched. Nonetheless, preweaning colostrum and milk nutrition has been demonstrated to influence first-lactation milk yield, although how much of this is a consequence of increased nutrient intakes and how much is a result of bioactive factors stimulating a mechanism of metabolic programming is unclear (Faber et al, 2005; Moallem et al, 2010; Soberon et al, 2012; van Amburgh et al, 2014; Andrews, 2016).
Hormone, cytokines, enzymes and growth factors
Of the many hormones (including leptin, relaxin, prolactin, oestradiol and cortisol), cytokines (such as IL-1β, IL-6, TNF-α and IFN-γ) and growth factors (including transforming growth factor) present in colostrum, the role of growth hormone, insulin and in-sulin-like growth factor (IGF) and their effects on somatotrophic axis function are probably the most researched. Insulin and IGF-1 concentrations within colostrum are high, the former around 20 and 100 times greater than that of serum and milk respectively, although concentrations of both are reduced by heat treatment (Mann et al, 2020; Baumrucker et al, 2021). They exert local effects within the intestinal lumen, promoting cell proliferation and villus growth resulting in improvements in intestinal absorptive capacity (Hammon et al, 2020). As a consequence, larger amounts of carbohydrates such as glucose are absorbed into the systemic circulation, resulting in greater endogenous production of IGF-1 and insulin. The improved activation of the somatotrophic axis promotes an anabolic state for the calf, which may maximise growth potential (Andrews, 2016; Hammon et al, 2020).
Trypsin-inhibiting enzymes within colostrum prevent the enteric digestion of immunoglobulins, but may also facilitate the persistence of Β-toxin, produced by Clostridium perfringens types B and C, which is trypsin sensitive. The inhibition of this enzyme may be rarely associated with enterotoxic disease in colostrum-fed calves, although this is more common in lambs (Duchesnes et al, 2006; Otter and Uzal, 2020).
Lactoferrin
Lactoferrin is a glycoprotein that plays a role in providing innate immunity. It acts to sequester iron, limiting its availability to pathogens that require the metal for metabolic processes (Kell et al, 2020). It also can enhance immune responsiveness, particularly natural killer cell activity, and prevent the entry of a virus into host cells. It is preserved during freezing and thawing, but is reduced by heat treatment (Lorenz, 2021; Robbers et al, 2021). Lactoferrin has achieved mixed results when evaluated as a supplement within milk, or as a treatment for diarrhoeic calves. Increases in average daily liveweight gain and reductions in morbidity have been described, although a randomised controlled trial failed to demonstrate any beneficial effect when used as a treatment for diarrhoea (Joslin et al, 2002; Pempek et al, 2019).
Oligosaccharides
Defined as carbohydrates with between two and ten covalently linked monosaccharides, the physiological purpose of oligosac-charides in bovine colostrum is considered to be non-nutritive, rather than an energy source (Zhang et al, 2021). Their main function has been associated with a prebiotic role, acting as a carbon source for beneficial bacteria as well as enhancing uptake of immunoglobulin in the small intestine (Park and Nam, 2015; Fischer-Tlustos et al, 2020), but they may also act as competitive inhibitors at the intestinal epithelial surface, preventing adhesion of pathogenic bacteria or viruses (Gopal and Gill, 2000).
Somatic cells
Epithelial cells, erythrocytes and leucocytes are the main somatic cells within colostrum (Blum, 2006). Approximately 106 maternal leucocytes, predominantly macrophages and monocytes (and to a lesser extent, T-lymphocytes), are contained within each millilitre of bovine colostrum (Gonzalez and Dus Santos, 2017; Lorenz, 2021). Similar to immunoglobulins, these cells are able to cross the intestinal barrier of the neonatal calf. They then migrate to the mesenteric lymph nodes, Peyer patches and spleen where they provide a memory function vs recognised antigens (Langel et al, 2015). They may also act as a source of CD4+ T cells responsible for B cell recruitment, neutrophil recruitment and enhanced phagocytic activity of macrophages, and influence the development of cellular and humoral immune responses to vaccination in neonatal calves (Langel et al, 2015; Meganck et al, 2016). It is important to note that these cells are destroyed or significantly reduced by freezing and heat treatment respectively, although the clinical significance of this appears to be minimal at present (Lorenz, 2021).
Transition milk
In the days following calving, the compositional characteristics of the mammary secretions change as colostrum production ceases while milk production increases. Commonly referred to as ‘transition milk’, it has been practically defined as the harvested milk from the subsequent six milkings after initial colostrum collection, by which time milk has usually reached the composition of saleable whole milk (Foley and Otterby, 1978). The benefits of feeding transition milk are re-emerging. Feeding for as little as 3 days promotes maturation of the small intestine, and longer-term feeding has been associated with improvements in dry matter intake, feed efficiency and daily liveweight gain (Pyo et al, 2020; Kargar et al, 2021). Transition milk feeding may also enhance mucosal immunity and encourage the development of a ‘friendly’ micro-biome, and has been associated with reductions in enteric disease (Hromádková et al, 2020; Kargar et al, 2020).
Interestingly, growth benefits have also been realised with short-term (3-day) feeding of milk replacer supplemented with colostrum replacer and it has been speculated, but not proven, that this may be the result of provision of bioactive factors (van Soest et al, 2020). It is important to consider that, in some circumstances, transition milk may contain antimicrobial residues, usually a consequence of antibiotic dry cow therapy. In these cases, there are drawbacks to transition milk feeding, as it has been associated with a shift in the intestinal microbiome and faecal shedding of antimicrobial-resistant bacteria (Firth et al, 2021). On this basis, it is advisable to only feed calves transition milk produced by cows that are not under an antibiotic milk withdrawal.
Call for practices to take part in our research project
Within our research group we are looking to recruit veterinary practices for a project entitled ‘Assessment of current colostrum feeding practices and extent of passive transfer in dairy herds in the United Kingdom’ as part of a PhD project by George Lindley. We would like to use serum total protein data routinely collected by practitioners alongside a short questionnaire to determine an estimate of the individual- and herd-level prevalence of failure of passive transfer within the UK, as well as to understand how dairy farmers are currently feeding colostrum. The results will allow benchmarking of herds to be performed on a national level, which we hope to be able to report to enrolled practices by the end of 2023. The success of the project is somewhat dependent upon recruitment of a large number of calves, farms and practices and we are enthusiastic about discussing the project with practices and farmers who might be keen to get involved. If the project is something that you might consider participating in, or if you would just like more information, please contact George by email: glindley2@rvc.ac.uk
Conclusions
Colostrum feeding of the neonate has critical importance for calf health, development and nutrition. It acts as a nutritional segue between in utero and milk feeding, providing energy and protein crucial for growth as well as required vitamins and minerals that have not passed through the placental circulation in appreciable quantities. Maternally-derived antibodies and maternal leuco-cytes provide passive immunity, while growth factors and hormones aid development. Components also act locally, stimulating development of the gastrointestinal epithelium as well as its microbiome, and inhibiting pathogen adhesion and survival. As the beneficial short- and long-term effects of non-nutritive factors within colostrum are further elucidated, consideration of these factors is likely to play a role when measuring colostrum quality in the future.
KEY POINTS
- Colostrum is rich in lipid, protein, vitamins and minerals, crucial for the provision of early life nutrition including essential factors which do not pass into the fetal circulation in significant quantities in utero.
- Immunoglobulins facilitate the transfer of passive immunity, alongside other immune cells such as maternal leucocytes, from the dam to the agammaglobulinaemic calf.
- Insulin-ike growth factors and insulin are present in high concentrations within colostrum and exert local effects that stimulate intestinal growth and activation of somatotrophic axis function.
- MicroRNAs regulate gene expression at the post-transcriptional level, promoting development of the intestinal epithelium as well as the immune system of the calf.
- Transition milk feeding provides an ongoing source of non-nutritive factors which may have local and systemic benefits to the calf.