The benefits of optimal colostrum provision for calves are well documented. Importantly, colostrum feeding results in transfer of passive immunity (TPI), by providing the initially agammaglobulinaemic neonatal calf with a source of immunoglobulins (of which greater than three quarters in colostrum is immunoglobulin G (IgG); McGrath et al, 2016). This enables an effective immune response when required. In addition to this predominant function, colostrum ingestion by the calf is known or suspected to have a range of other benefits, including provision of vital nutrients (energy and protein) at the start of life, improved gut development, local gut immunity, establishment of the gut microbiome and improved glucose metabolism (Hammon et al, 2020). Consequently, calves ingesting adequate colostrum experience reduced incidence of morbidity and mortality (primarily respiratory and enteric diseases; Donovan et al, 1998; Raboisson et al, 2016; Glover et al, 2019), and can have improved growth rates (Windeyer et al, 2014) and future milk yields (DeNise et al, 1989). While many of the benefits of colostrum intake are also under the influence of a multitude of other factors, acquisition of a functional immune system providing protection against disease during early life is highly dependent on colostrum provision during the first hours of life; with regards to immunity of young calves, there is no substitute for adequate colostrum. This article will describe the factors that are important in ensuring optimal ingestion and intestinal absorption of immunoglobulin G by the neonatal dairy calf, as well as methods used to assess TPI, with the aim of providing a framework for reducing the incidence of failure of transfer of passive immunity (FTPI).
Failure of transfer of passive immunity definition
The definition of FTPI has often been stated to be a serum IgG concentration of less than 10 mg/ml. While calves with serum IgG concentration below this cutpoint are consistently found to be at greater risk of mortality when compared with calves with higher concentrations, close examination of research indicates that this cutpoint is likely to be an overly simplistic view of the concept of adequate TPI. Instead, it would appear that calves may further benefit from serum IgG concentrations considerably higher than 10 mg/ml in terms of reduced risk of mortality (Urie et al, 2018; Lombard et al, 2020), and that in order to be optimally protected against morbidity (i.e. regardless of whether death occurs), cutpoints of 15 mg/ml, or even 25 mg/ml are more appropriate (Furman-Fratczak et al, 2011; Windeyer et al, 2014; Urie et al, 2018).
Incidence of failure of transfer of passive immunity
Recent studies provide some evidence of FTPI incidence on UK dairy farms; the percentage of calves with inadequate circulating IgG has been found to be 20.7% (492 calves on 11 farms in South-east England; Johnson et al, 2017), 26% (444 calves on 7 farms in Northwest England; MacFarlane et al, 2015) and 48% (414 calves on 75 farms in Wales; Atkinson 2015) (Figure 1). These studies used a serum IgG concentration of 10 mg/ml for defining FTPI; it is not unreasonable to assume that between a quarter and a half of UK dairy calves suffer FTPI using even this modest definition, and that, in light of recent research suggesting that 10 mg/ml is a conservative target, many more dairy calves could be better protected against disease if colostrum provision were improved.
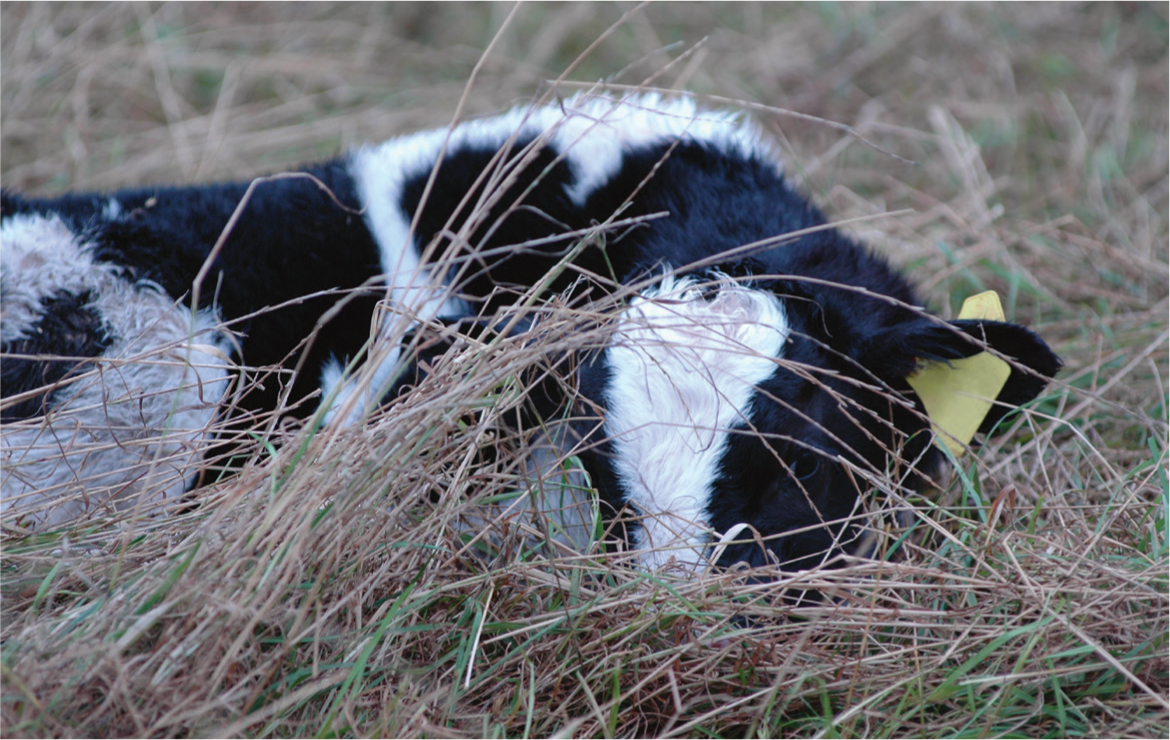
Apparent efficiency of absorption
In order to achieve adequate circulating IgG, a neonatal calf must consume a sufficient mass of immunoglobulins, followed by effective uptake of those immunoglobulins from the intestines. The mass of IgG consumed is a product of the volume of colostrum ingested, and the concentration of IgG within that colostrum. The proportion of consumed IgG that is absorbed from the intestines (apparent efficiency of absorption; AEA), is determined, in part, by the age of the calf; a reduction in AEA ensues from birth to approximately 24 hours old as a result of a progressive decrease in the capacity of enterocytes to absorb complex proteins (‘gut closure’). Extending the interval between birth and colostrum ingestion therefore leads to reduced serum IgG concentration (Shivley et al, 2018). While gut closure is often thought of as proceeding at a constant rate over the first day of life, some studies have found that AEA reduces more slowly during the first 4–12 hours of life (Osaka et al, 2014; Halleran et al, 2017); there is at least a suggestion that IgG absorption decreases exponentially as the birthcolostrum interval is extended, or that complete gut closure is delayed slightly if the interval from birth to feeding is delayed (Stott et al, 1979), meaning that short delays (4 to 6 hours) between birth and colostrum-feeding are unlikely to significantly reduce AEA. AEA has been found to be in the range of 15 to 50% (Besser et al, 1985; Elizondo-Salazar and Heinrichs 2009; Godden et al, 2009; Conneely et al, 2014; Fischer et al, 2018). Thus, to achieve a serum IgG concentration of 20 mg/ml, an average weight Holstein calf is required to consume approximately 200 g of IgG shortly after birth. It appears possible to overwhelm the capacity of the intestines to absorb IgG (Besser et al, 1985; Chigerwe et al, 2008; Conneely et al, 2014; Saldana et al, 2019), leading to a ‘law of diminishing returns’; AEA reduces as colostrum quality increases. Providing more than 200g of IgG at the first colostrum feeding is of questionable benefit (Besser et al, 1991) if the first feeding occurs within a short time of birth. Finally, there is some evidence of an association between dystocia or neonatal acidosis, and reduced AEA (Beam et al, 2009; MacFarlane et al, 2015), although this evidence is inconsistent (Weaver et al, 2000; Sangild 2003).
Colostrum quality
IgG concentration is properly measured in a laboratory, but a practical, cheap and very accurate assessment can be made on farm using the Brix refractometer. The colostrometer, which measures colostrum density, has largely been superseded because of often low correlation with IgG concentration, even when measuring colostrum at consistent temperature (Pritchett et al, 1994; Morin et al, 2001), and because it is a fragile glass instrument. Using refractometry, colostrum with at least 23% Brix has a high probability of having an IgG concentration of at least 50 g/litre (the current industry standard for ‘good quality’ colostrum), while samples below 18% Brix are predicted to be below this standard (Buczinski and Vandeweerd, 2016). The association between percentage Brix and colostral IgG has been found to be linear, but the correlation between the two is imperfect, meaning that it is impossible to equate a refractometer reading to a precise IgG concentration (Bartier et al, 2015). Nevertheless, an estimate of colostrum quality, beyond ‘good’ (>=50 g/litre IgG) or ‘bad’ (<50 g/litre IgG) can be made, and interested readers are referred to Bartier et al (2015). Colostrum quality varies considerably, with reported minimum and maximum values, expressed as grammes per litre of IgG, in the single- and triple-figures respectively (Dunn et al, 2017; Barry et al, 2019; Johnsen et al, 2019). In a UK study, 37% of colostrum samples of dairy cows were found to be sub-standard (<22% Brix; MacFarlane et al, 2015). As a consequence, calves receiving colostrum of unmeasured IgG concentration may ingest very variable masses of IgG. Measurement of colostral IgG concentration by farmers, using a refractometer, is therefore an essential step in combatting FTPI. By routinely measuring colostrum quality, producers may aim to feed a target mass of IgG (e.g. 200 g), rather than aiming to feed a target volume of colostrum. Several factors are known to influence colostrum IgG concentration (Figure 2). Dams of higher parity, especially cows of third or higher parity, produce higher quality colostrum (Conneely et al, 2013; Silva-del-Río et al, 2017; Shivley et al, 2018). Volume of colostrum produced by a cow, prior to calving, is possibly negatively associated with IgG concentration (Pritchett et al, 1991; Zentrich et al, 2019). Subsequent dilution of colostrum by the initiation of lactation at the point of calving further reduces IgG concentration (Morin et al, 2010), although this may not result in a significant change in the quality of the harvested colostrum unless the delay between calving and first milking is considerable, for example 9–12 hours (Conneely et al, 2013; Dunn et al, 2017). In any case, the highest importance should be placed on the mass of IgG consumed by the calf; cows producing a high volume of colostrum may produce a large total mass of IgG, constituting several effective calf-doses of colostrum (Baumrucker et al, 2010). Very short (<28 days) dry periods, or omitted dry periods, are likely to result in reduced colostrum quality (Rastani et al, 2005; Mayasari et al, 2015; Gavin et al, 2018), but no difference in colostrum quality was observed between dams of 40-day and 60-day dry periods (Shoshani et al, 2014). Seasonal variation in colostrum quality has been demonstrated (Conneely et al, 2013; Gavin et al, 2018; Johnsen et al, 2019), as has an effect of prepartum heat-stress (Nardone et al, 1997; Shivley et al, 2018), although overall these environmental factors appear to have an inconsistent or minor association with colostrum quality (Pritchett et al, 1991; Morin et al, 2010) and are often beyond practical control. While feeding prepartum diets with excessive energy content has been found to reduce colostrum IgG concentration (Mann et al, 2016), the influence of prepartum nutrition (energy and protein provision) on colostrum quality is still an area of ongoing research.
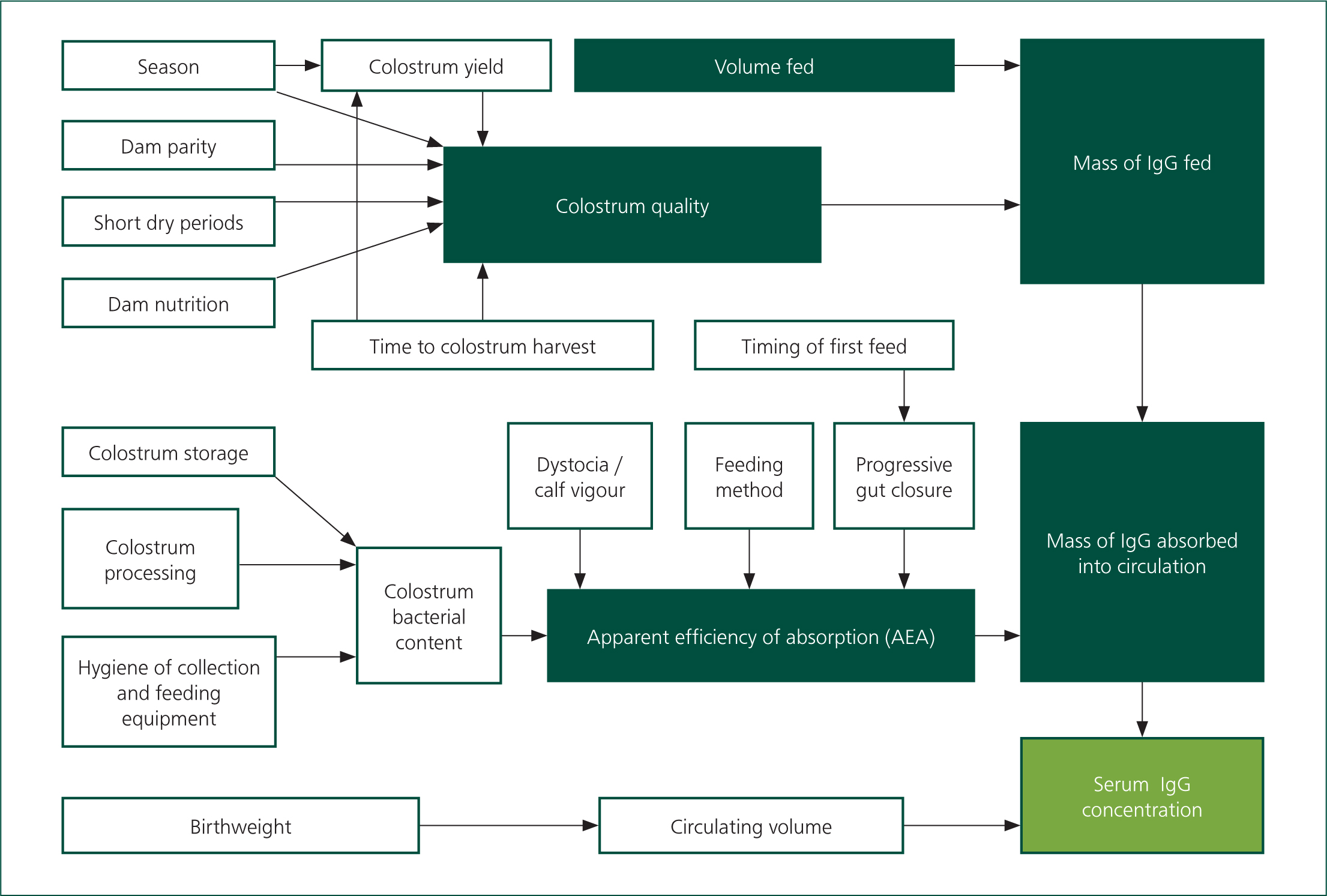
Colostrum bacterial counts
Presence of bacteria in colostrum reduces AEA and therefore leads to lower circulating IgG. The mechanism for this effect is unclear (Elizondo-Salazar and Heinrichs, 2009), but has been postulated to be because of direct binding of colostral IgG by bacteria, or by bacterial inhibition of uptake of IgG by enterocytes. Regardless, minimising bacterial counts in colostrum is highly important. Industry targets for colostrum total bacterial count (TBC) and coliform count (at the point of ingestion) are <100000 and <10000 colony-forming units (cfu) per millilitre, respectively, although these targets are somewhat arbitrary; AEA has been shown to be inhibited at TBC considerably less than 100000 cfu/ml (Johnson et al, 2007), indicating that reducing TBC to below 100000 cfu/ml is beneficial. The overwhelming source of bacteria in colostrum is not the mammary gland itself, but the environment to which it is exposed immediately after leaving the teat (teat skin, milking machine), and the container into which it is collected (Stewart et al, 2005). A recent UK study (Hyde et al, 2020) discovered that approximately one-third of colostrum samples had a TBC above 100000 cfu/ml at the end of the harvesting process (i.e. in the container used for collection), and found that thorough premilking teat preparation, and disinfection before each use of all equipment used for collection, is imperative for minimising TBC. Cleaning and disinfection of feeding equipment (e.g. ‘stomach’ tubes) before each use is also important. Bacterial multiplication within colostrum stored at ambient temperatures will further increase TBC, especially during warmer months; the common practice of keeping colostrum in (often non-sterile) containers in the dairy is to be discouraged. Colostrum should be stored (refrigerated for a number of hours, or frozen as soon as possible) if not fed to calves promptly.
Heat treatment
Heat treatment of colostrum (60°C for 30–60 minutes) drastically reduces bacterial counts, thereby improving AEA (Gelsinger et al, 2015) and ultimately increasing circulating IgG (Johnson et al, 2007; Elizondo-Salazar and Heinrichs, 2009; Donahue et al, 2012; Gelsinger et al, 2014; Cummins et al, 2017; Shivley et al, 2018). Heat treatment results in the further benefit of significantly reducing the numbers of some important pathogens, for example Escherichia coli, Salmonella spp., and Mycoplasma bovis. Mycobacterium avium subspecies paratuberculosis (MAP), the causal organism in Johne's disease, is relatively more able to withstand high temperatures, but heating to 60°C for 60 minutes (a common protocol in the UK), will reduce the number of MAP organisms to very low levels (Godden et al, 2006). Use of higher temperatures (>60°C) or durations longer than 60 minutes will eliminate more bacteria, but will also result in other changes to colostrum, including significant denaturation of IgG (McMartin et al, 2006; Elizondo-Salazar et al, 2010) and changes in colostrum consistency. Treatment at 60°C for 60 minutes does reduce IgG concentration, usually by less than 10% or even less than 5% (McMartin et al, 2006; Johnson et al, 2007; Elizondo-Salazar and Heinrichs, 2009; Donahue et al, 2012; Gelsinger et al, 2014); this small loss of IgG is more than compensated for by the improved AEA which results from elimination of bacteria. It is also worth noting that heat treatment destroys colostral leukocytes (as does freezing of colostrum), but while it is thought that transfer of leukocytes from dam to calf may play some role in calf immunity, no evidence exists that feeding leukocyte-free colostrum has any significant effect on calf health (Johnson et al, 2007; Meganck et al, 2014; Godden et al, 2019).
Method of colostrum feeding
Natural suckling of colostrum is commonly found to increase the probability of FTPI (Trotz-Williams et al, 2008; Lora et al, 2018), even when calves are given assistance to suck (Besser et al, 1991). Moreover, supervision of suckling is time-consuming and may be overlooked as a result. Intervention through feeding colostrum via a bottle with a teat or, as is more common in the UK, via an oesophageal (‘stomach’) tube should be recommended. The oesophageal groove does not close when calves are ‘stomach tubed’, leading to colostrum entering the ruminoreticulum. This potentially extends the interval between feeding and the arrival of colostrum at the intestines, but because of the small capacity of the neonatal ruminoreticulum, unless very small volumes (below 1.5 litres) are being fed, the majority of colostrum will rapidly pass into the abomasum anyway (Lateur-Rowet and Breukink 1983; Godden et al, 2009; Desjardins-Morrissette et al, 2018). Studies have found no difference in circulating IgG between calves fed using a teat or using an oesophageal tube (Besser et al, 1991; Chigerwe et al, 2012), as long as an adequate volume (for example 3 litres) is being fed (Godden et al, 2009). Feeding of colostrum using either method (bottle or tube) is acceptable, although some consider ‘stomach tubing’ calves to be invasive, and farmers using this method must be properly trained in use of an oroesophageal feeder in order to avoid rare complications (e.g. inhalational pneumonia).
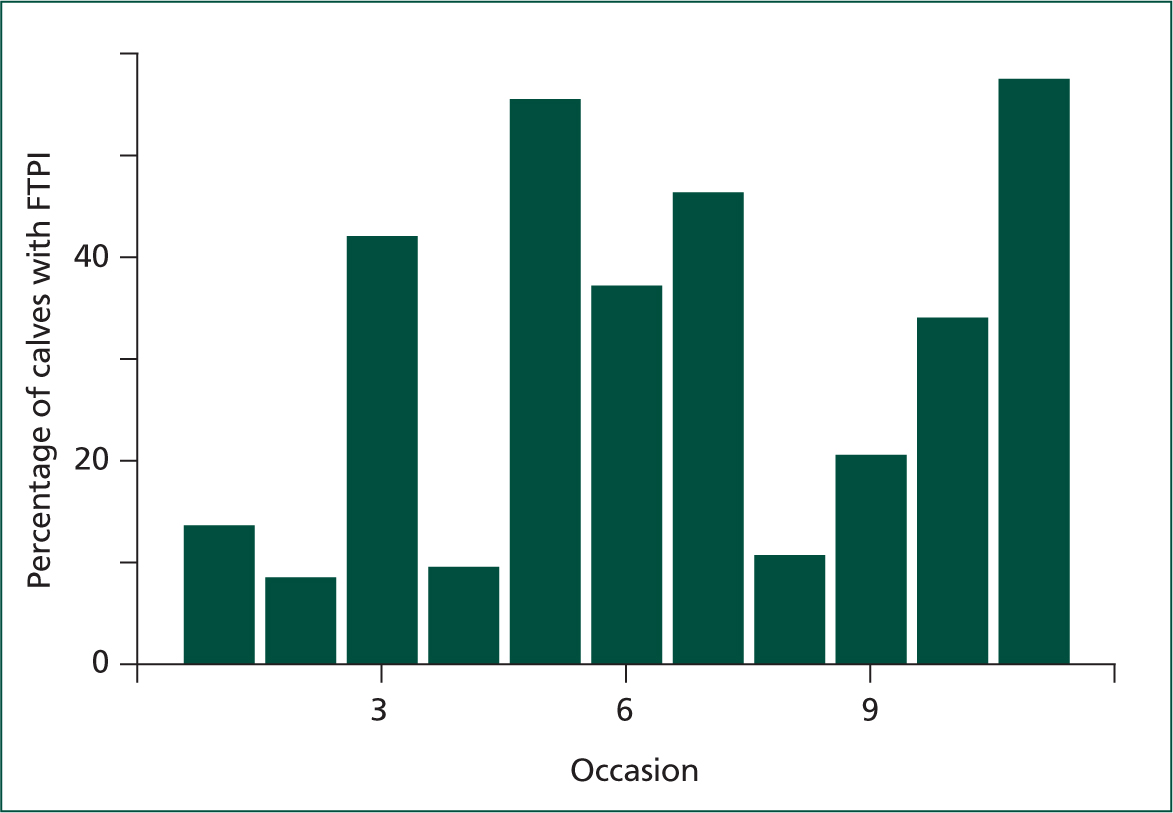
Assessment of transfer of passive immunity
Accurate measurement of a calf 's circulating IgG concentration can be achieved with radial immunodiffusion (RID) by a reference laboratory. However, commercial availability of this test is limited. A cheap and practical alternative for the on-farm or in-practice situation, is the measurement of blood total protein concentration, either serum total protein (STP) or plasma total protein (PTP). A considerable proportion of blood protein comprises gammaglobulins. Therefore, circulating protein concentration can be used as a proxy measure of circulating IgG. Protein concentration can be measured in serum or plasma with a refractometer (which may give a reading as %Brix or as total protein, neither of which is superior). Blood sampling should be done between 24 hours and 9 days of age (Trotz-Williams et al, 2008; Wilm et al, 2018). Dehydration, for example as a result of illness, elevates STP, therefore un-well calves should not be sampled. Refractometer measurements of STP are well (>80%) correlated with serum IgG concentration (Deelen et al, 2014; Hernandez et al, 2016). However, this correlation is imperfect, and so any result of a refractometer measurement of STP will correspond to a small range of potential values of IgG concentration. Studies have found various optimal cutpoints for determining, for example, which value of STP serves best as an indicator of FTPI (<10 mg/ml serum IgG; Tyler et al, 1996; Calloway et al, 2002; Morrill et al, 2013; Deelen et al, 2014; Elsohaby et al, 2015; Shivley et al, 2018; Urie et al, 2018). In light of this, and of new knowledge elucidating the benefits of circulating IgG far above a concentration of 10 g/litre, this author recommends the use of 5.5 g/dl STP (or 8.5% Brix), as a good starting point for determining FTPI. It is worth noting that, if feeding pasteurised or powdered colostrum, these cutpoints should be adjusted. The relationship between STP and serum IgG has been shown to be altered following feeding of heat-treated colostrum, possibly as a result of reduced intestinal uptake of non-IgG colostral proteins. If calves are fed pasteurised colostrum, a cutpoint approximately 0.2 g/dl lower is appropriate. (Elizondo-Salazar and Heinrichs, 2009; Gelsinger et al, 2015). Regarding colostrum replacer (powdered colostrum), the correlation between STP and serum IgG is unknown because of the variability in protein composition of different products, and therefore STP monitoring is difficult in this situation. As a result of the inherent uncertainty of the true IgG concentration when measuring STP, assessment of prevalence of FTPI within the herd is more useful than the testing of small numbers of individual calves. Previous recommendations have been made for the sampling of a small number, for example 12, calves on a farm, the number of which fall below a cutpoint (STP or %Brix) indicating if FTPI is problematic on a farm (McGuirk and Collins, 2004). While this approach is easy to implement, FTPI is complex (Figure 1); differences in factors influencing FTPI prevalence may be present between groups of calves and over time. For example, weekly STP testing by the author of small numbers of calves on one dairy farm (Figure 2) revealed that the proportion of calves falling below a defined threshold is highly variable, even in the face of an apparently unchanging colostrum management policy. Any conclusions drawn from occasionally sampling a small number of calves must be viewed with appropriate scepticism, and regular testing of calves, for example at routine fertility visits, is essential.
Conclusion
The incidence of FTPI remains problematic on many dairy farms. With a solid understanding of the many factors influencing FTPI, veterinarians and consultants should be well placed to advise on optimising colostrum management, thereby maximising calf health and welfare.
KEY POINTS
- For monitoring of transfer of passive immunity (TPI), or if it is suspected that TPI is not optimal, calves 1–9 days old should be blood sampled on a routine basis, for example during fertility visits. Drawing conclusions from samples taken on an occasional basis from a small number of calves is inadequate. The effect of any changes made to colostrum protocol can be further monitored in the same way.
- Most of the factors that influence colostrum quality are impractical to control, and therefore colostrum quality can only be monitored through the use of refractometers by farmers. It should be remembered that, while refractometers can be used for estimation of colostrum quality, feeding colostrum of a certain quality to a calf is not the point. Instead, the aim should be to administer 200 g of IgG to every calf, regardless of volume or quality. Progressive farmers can be encouraged to assess, store and feed colostrum doses in terms of total IgG mass rather than IgG concentration.
- Research finds that bacterial contamination of colostrum is often considerable at the point of feeding, and that this will have a significant impact on uptake of IgG by enterocytes. Pre-milking teat disinfection, and disinfection of colostrum collection and feeding equipment is paramount. Assessment of colostrum bacterial counts can be made by a commercial laboratory. Total bacterial counts (TBCs) and coliform counts of below 100000 and 10000 cfu/ml, respectively, are often quoted as targets, but these targets may be too high as lower counts will also result in inhibition of IgG uptake.
- Heat-treatment of colostrum (e.g. 60°C for 60 minutes) drastically reduces colostral bacterial counts, thereby improving apparent efficiency of absorption; pasteurisation should be implemented on farms wherever possible.
- Calves should be fed colostrum by ‘stomach tube’ or by bottle wherever possible; reliance on suckling for adequate colostrum provision is often unsuccessful.