Bovine respiratory disease (BRD) is a complex, multifactorial syndrome that, despite substantial research (Fulton, 2009), remains a significant problem costing the UK cattle industry an estimated £50 million annually (Statham et al, 2018). An average BRD prevalence of almost 50% has been reported in pre-weaned dairy heifers (Johnson et al, 2017) and almost 70% of UK cattle farmers experience BRD in their herds with nearly 50% reporting animal losses attributed to BRD (Baxter-Smith and Simpson, 2020).
Bovine respiratory disease arises due to a combination of animal (host) factors, environmental factors and pathogen factors (Figure 1). Animal factors are those that affect individual susceptibility to infection such as immune status or stress. Environmental factors affect the susceptibility of individuals or groups to infection such as ventilation, over-stocking or a high degree of pathogen challenge in the environment. Pathogen factors are features of different pathogens that increase their pathogenicity — for example, virulence factors such as leukotoxin production.
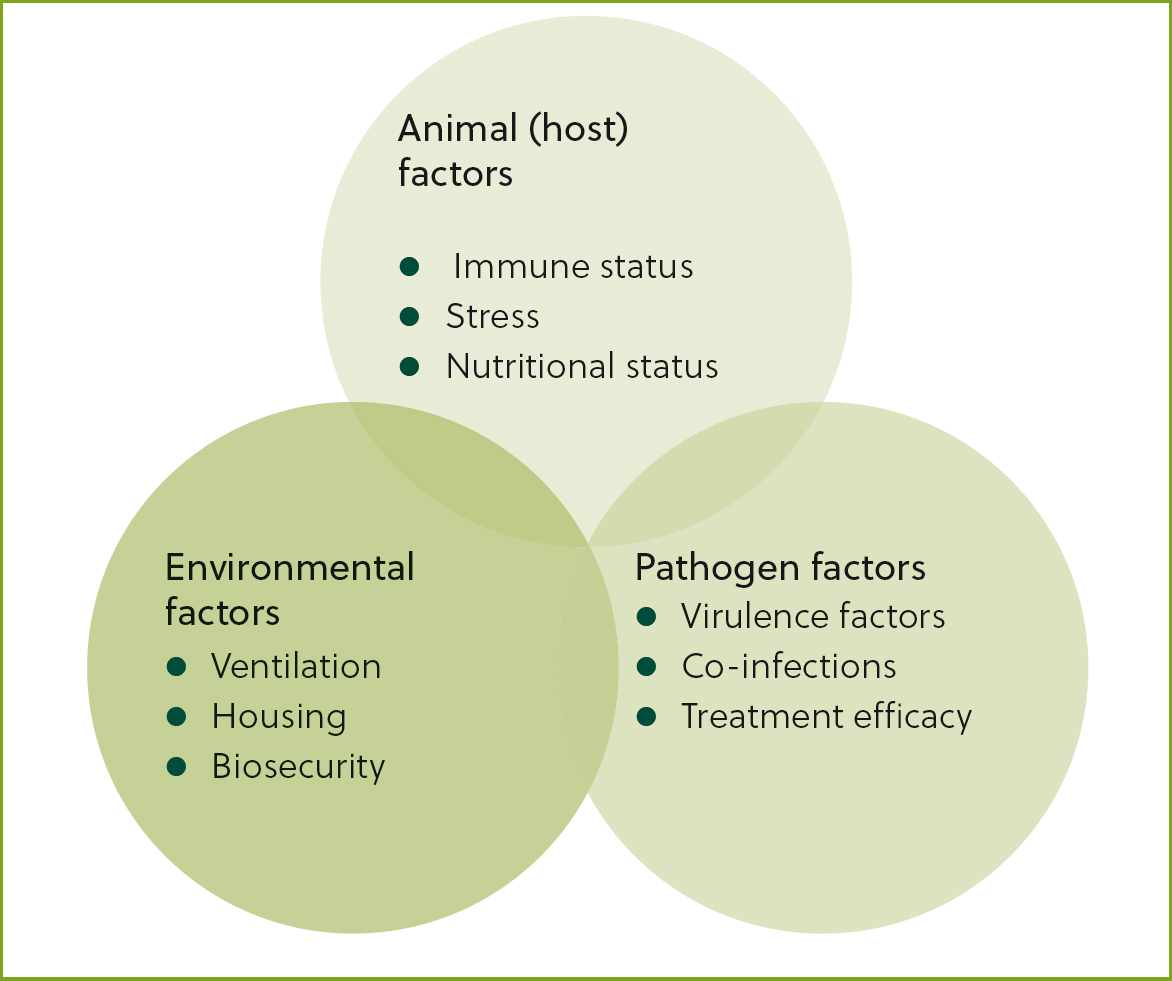
Pathogens implicated in bovine respiratory disease
It is not usually possible to determine the aetiology of BRD based on clinical examination alone; signs consistent with pneumonia are often observed irrespective of the causative agent. Both bacterial and viral pathogens are implicated, and in many cases, disease occurs due to co-infection with more than one pathogen, further complicating diagnosis.
Histophilus somni, Mannheimia haemolytica, Pasteurella multocida, and Mycoplasma bovis are widely considered to be the primary bacteria involved in BRD. While all have been isolated from healthy animals (Griffin et al, 2010; Constable et al, 2017) — and likely contribute to the bovine upper respiratory tract microbiome (Holman et al, 2015; Centeno-Martinez et al, 2022) — they are also frequently isolated from diseased calves. In uncomplicated cases where secondary bacterial infection is not present viral BRD caused by PI-3 or BRSV typically presents as broncho-interstitial pneumonia; however interlobular emphysema may also be seen in BRSV infections (Ellis, 2010; Makoschey and Berge, 2021).
Respiratory pathogens such as bovine coronavirus, bovine adenovirus, bovine rhinitis virus and bovine influenza virus are less commonly isolated and have traditionally been considered to be minor BRD pathogens. As our understanding of BRD pathogenesis develops, the role of these viruses in the BRD complex is being re-evaluated (Murray et al, 2016) and it is possible that in the future they may grow in importance.
Major bacterial pathogens
Pasteurella multocida has been found to be present in up to 60% of healthy calves in some herds (Angen et al, 2009; Gaeta et al, 2017), suggesting it is a commensal respiratory tract inhabitant in some calves. However, this is not a consistent finding with P.multocida being isolated up to twice as frequently from diseased calves (compared to healthy calves) in other herds (Angen et al, 2009; Griffin et al, 2010). Furthermore, last year P.multocida was the most commonly diagnosed cause of BRD in Great Britain (APHA, 2023); accordingly, P.multocida is considered an important bovine respiratory pathogen.
Bovine respiratory disease attributed to Mannheimia haemolytica infection is often seen in weaned calves and adult cattle, particularly in beef systems, and typically presents as a fibrino-haemorrhagic pneumonia with associated pleuritis. A fibrinous pericarditis may also be present. Postmortem findings associated with M.haemolytica infection include widened interlobular spaces, often with gelatinous, yellowish-coloured material.
Mannheimia Haemolytica is a commensal inhabitant of the bovine upper respiratory tract and an opportunistic pathogen of the lower respiratory tract; co-infection with respiratory viruses is thought to have an important role in facilitating M.haemolytica colonisation of the lower respiratory tract and can also enhance virulence (Jones and Chowdhury, 2010). Of the 12 M.haemolytica serotypes, serotypes A1, A2, and A6 are most commonly isolated (Mason et al, 2022). Historically, M.haemolytica serotype A2 has been considered a non-pathogenic commensal of the bovine respiratory tract; however, recent studies have isolated M.haemolytica from animals affected with clinical BRD, casting doubt on this view (Arnal et al, 2021; Mason et al, 2022), but further work is needed to fully understand the importance of M.haemolytica serotype A2 in the BRD complex.
Histophilus somni is a gram-negative bacteria associated with several clinical syndromes including respiratory disease (Figure 2). Pleuritis and fibrinopurulent pneumonia are typically observed in the respiratory form, with large amounts of fibrin deposits often being present at postmortem examination (Griffin et al, 2010). In the past two decades, H.somni has been recognised as a major respiratory pathogen in cattle and is being diagnosed with increasing frequency. For example, between October and December 2023, pneumonia attributed to H.somni infection was the third most common postmortem diagnosis made in Great Britain, with almost twice as many cases being diagnosed compared to the average number of diagnoses made in the same time period (October –December) over the preceding decade (APHA, 2023).
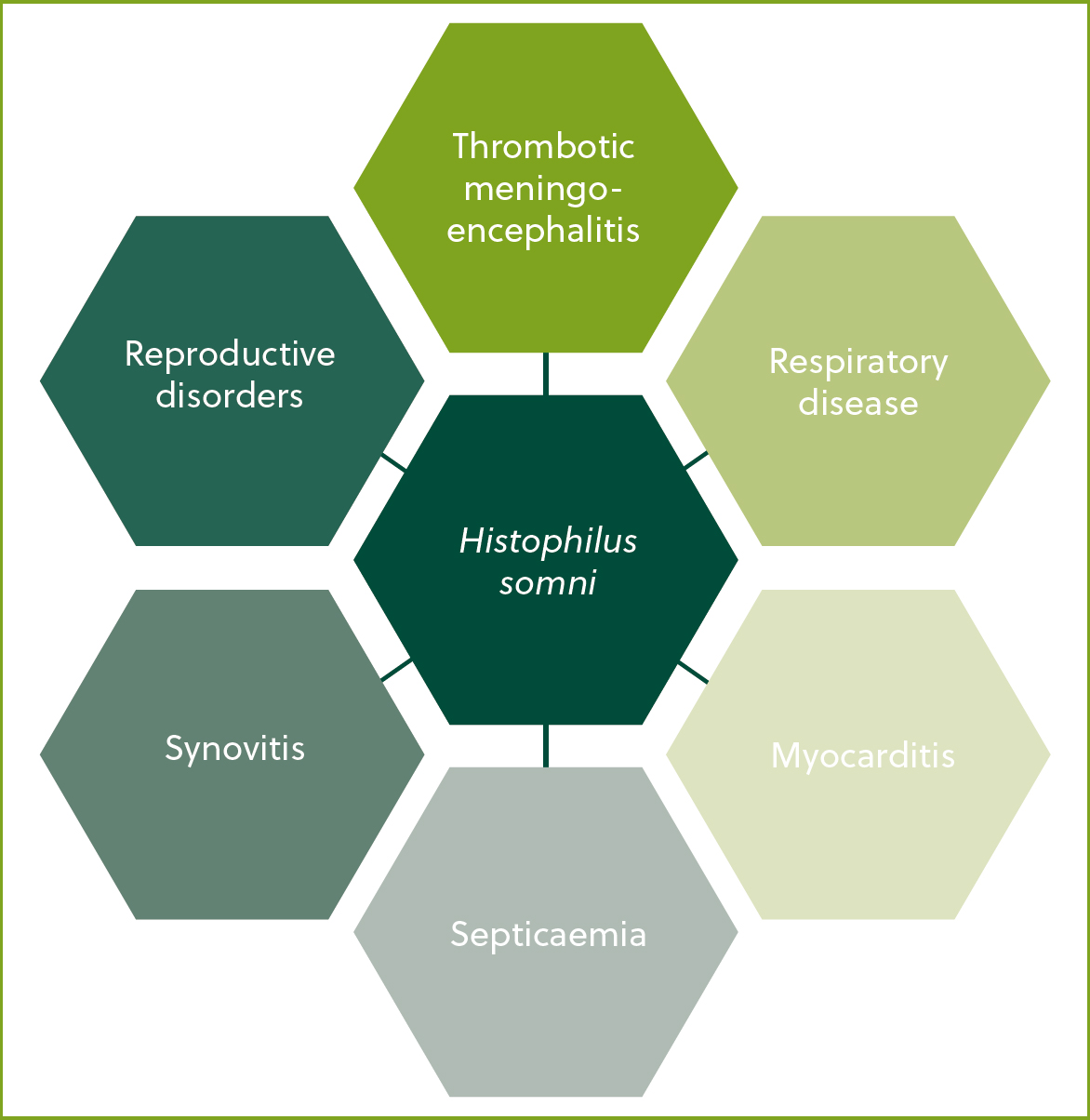
Mycoplasma bovis is one of several bovine mycoplasma species and is associated with a number of clinical presentations including mastitis, otitis media, arthritis, bronchopneumonia, keratoconjunctivitis, and meningitis (Maunsell et al, 2011). Calves affected by the respiratory form of M.bovis may present with coughing and a nasal discharge, and a caseonecrotic bronchopneumonia is commonly observed postmortem (Caswell et al, 2010). Calves affected with BRD caused by M.bovis infection may also present with a head tilt (indicative of otitis media) and swollen joints (indicative of arthritis).
Major viral pathogens
Parainfluenza-3 virus and BRSV are related viruses that are both capable of penetrating the respiratory mucus barrier (Makoschey and Berge, 2021), compromising mucosal immunity and facilitating infection by other BRD pathogens. Both PI-3 virus and BRSV are common co-infections with other BRD pathogens but in uncomplicated cases clinical signs include rhinitis, pyrexia and coughing and are variable in severity, although severe disease is more commonly associated with BRSV infection (Brodersen, 2010; Ellis, 2017).
Bovine herpesvirus-1 is the causative agent of infectious bovine rhinotracheitis (IBR). In the absence of secondary bacterial infection, clinical signs are consistent with upper respiratory tract disease and include coughing, nasal discharge, conjunctivitis and inflammation of the nose (IBR is sometimes referred to as ‘red nose’). Similar to PI-3 virus and BRSV, infection with BHV-1 can predispose cattle to secondary bacterial infection with BRD pathogens, particularly M.haemolytica (Jones and Chowdhury, 2010).
Prevention of bovine respiratory disease
Optimisation of individual and herd defences against BRD pathogens, together with minimising pathogen exposure is fundamental to BRD prevention. Effective colostrum management has a key role in establishing robust immune responses in newborn calves and immunity to specific pathogens can be established using vaccination. Optimising nutrition, housing and preventing disease incursion and spread by adopting responsible biosecurity practices are also important for BRD prevention.
Colostrum management
Effective transfer of passive immunity is a crucial aspect of BRD prevention and can be aided by the establishment of a systematic colostrum management protocol. Calves should be fed a volume of colostrum equivalent to 10–12% of their body weight; for most Holstein calves this is approximately 4 L (Godden et al, 2019). Conventional advice is to ensure calves have consumed colostrum by four to six hours after birth, but the steady decline in efficiency of immunoglobulin absorption that occurs after birth means that absorption is significantly lower at six hours old compared to birth (Fischer et al, 2018). Accordingly, some authors are now suggesting that six hours is too long a delay and calf-rearers should aim to feed colostrum within two hours of birth (Godden et al, 2019). Feeding colostrum using a bottle is preferable to an oesophageal tube as suckling from a teat stimulates oesophageal groove closure, directing colostrum to the abomasum. However, when an adequate volume of colostrum is consumed, the feeding method has not been found to affect passive transfer (Desjardins-Morrissette et al, 2018), therefore, if bottle feeding is not possible or inadequate, the use of an oesophageal tube can be considered an efficient method of ensuring calves consume enough colostrum with minimal effect on transfer of passive immunity.
Immunoglobulin content of colostrum is highest at calving and declines thereafter, with one study finding that colostrum IgG concentration decreased by almost 4% for every hour after calving that harvest was delayed (Morin et al, 2010). Similarly, another study found that IgG concentration of colostrum harvested two hours after calving was significantly higher than colostrum harvested six or more hours after calving (Moore et al, 2005). As such, colostrum should be harvested as soon as possible after calving – ideally within two hours.
Vaccination
Vaccination aims to enhance herd immunity and increase individual resistance to BRD pathogens (Sherwin and Down 2018). Several BRD vaccines are available in the UK, most of which are multivalent (Table 1) (National Office of Animal Health, 2024; Veterinary Medicines Directorate, 2024). The majority of available respiratory vaccines are live, modified-live, or inactivated vaccines but a product containing a recombinant-protein component has recently been developed (Divence, Hipra), although in the UK it is currently only registered for use in Northern Ireland (Veterinary Medicines Directorate, 2024). Vaccines targeted to BRD pathogens may include a combination of types within the same product (Table 1).
Manufacturer | Vaccine | Targeted pathogen(s)/diseases | Method of administration | Vaccine type |
---|---|---|---|---|
Boehringer Ingelheim | Bovalto Respi 3 | BRSV, PI-3, M.haemolytica (serotype A1) | Injection | Inactivated |
Bovalto Respi 4 | BRSV, PI-3, BVD, M.haemolytica (serotype A1) | Injection | Inactivated | |
Bovalto Respi Intranasal | BRSV + PI-3 | Intranasal | Modified live | |
Hipra | Divence IBR Marker live (NI only) | IBR | Injection | Live (IBR-gE gene deleted) |
Divence Penta (NI only) | BRSV, PI-3, IBR, BVD | Injection | Modified-live (BRSV), Live (IBR-gE deleted), inactivated (PI-3), recombinant-protein (BVD) | |
Divence Tetra (NI only) | BRSV, PI-3, BVD | Injection | Modified-live (BRSV), inactivated (PI-3), recombinant-protein (BVD) | |
NASYM | BRSV | Injection or intranasal | Modified-live | |
Hiprabovis SOMNI/Lkt | M.haemolytica (serotype A1) and H.somni | Injection | Inactivated | |
Hiprabovis IBR marker live | IBR | Injection | Live (IBR-gE gene deleted) | |
MSD Animal Health | Bovilis Nasalgen | Coronavirus | Intranasal | Modified-live |
Bovilis Intranasal RSP | BRSV + PI-3 | Intranasal | Live | |
Bovilis IBR Marker live | IBR | Injection or intranasal | Live | |
Bovilis IBR Marker inactivated | IBR | Injection | Inactivated | |
Bovilis Bovipast RSP | BRSV, PI3, M.haemolytica (serotypes A1 and A6) | Injection | Inactivated | |
Zoetis | Rispoval RS | BRSV | Injection | Modified-live |
Rispoval 2 | BRSV + PI-3 | Injection | Live | |
Rispoval 3 | BRSV, PI-3, BVD | Injection | Modified live (BRSV and PI-3) and inactivated (BVD) | |
Rispoval 4 | BRSV, IBR, PI-3, BVD | Injection | Modified live (BRSV and PI-3) and inactivated (IBR and BVD) | |
Rispoval RS+PI3 | BRSV + PI-3 | Intranasal | Live | |
Rispoval Pasteurella | M.haemolytica (serotype A1) | Injection | Inactivated | |
Rispoval IBR Marker live | IBR | Injection | Live | |
Rispoval IBR Marker inactivated | IBR | Injection | Inactivated | |
Protivity | M.bovis | Injection | Modified-live |
BRSV = bovine respiratory syncytial virus. PI-3 = parainfluenza virus-3. BVD = bovine viral diarrhoea. IBR = Infectious bovine rhinotracheitis. NI = Northern Ireland. 1. Data summarised from the NOAH compendium (NOAH 2024) and the Veterinary Medicines Directorate product information database (Veterinary Medicines Directorate, 2024) and is correct at the time of writing. The author accepts no responsibility regarding the use of these products; readers are recommended to refer to relevant product datasheets before administration.
Live and modified-live vaccines contain an attenuated version of the target pathogen that can replicate but is not capable of causing disease, although reversion to virulence can occur (Jorge and Dellagostin, 2017). By contrast, inactivated (killed) vaccines contain an inactivated version of the target pathogen that cannot replicate and, therefore, cannot revert to virulence. As such, inactivated vaccines may be safer than live or modified-live vaccines, but the immune response elicited is primarily humoral and more than one dose is required (Chamorro and Palomares, 2020). In comparison, live or modified-live vaccines stimulate both humoral and cell-mediated immune responses — offering more complete and longer-term protection (Chamorro and Palomares, 2020). To manufacture recombinant protein vaccines, a piece of DNA coding for specific proteins is transferred from the target pathogen into cells of a host microorganism (such as yeast). When these cells replicate, the host microorganism reproduces the pathogen protein, which is purified and used to create a vaccine. Similar to inactivated vaccines, pathogen replication cannot occur so there is no risk of reversion to virulence, the immune response elicited is primarily humoral and an adjuvant is typically needed, which can sometimes result in vaccine reactions (Jorge and Dellagostin, 2017).
Housing and biosecurity
Thermoregulation in environmental temperatures outside of a calf 's thermoneutral zone (10-15°C for calves under 2 weeks of age and 6–10°C in older calves) requires energy expenditure, and very high or low temperatures can result in heat or cold stress respectively. In wet, windy conditions calves’ lower critical temperature (the environmental temperature below which energy is required to maintain body temperature) increases (Roland et al, 2016), meaning that calves may suffer from cold stress at higher environmental temperatures than in dry conditions. Colder environmental temperatures are associated with increased calf mortality (Hyde et al, 2020); therefore, it is important that housing is well-designed to keep calves warm and dry. As perhaps might be expected, calves show a preference for soft, dry bedding (Camiloti et al, 2012). Using a deep layer of dry straw to bed calf pens not only meets this preference (contributing to positive welfare), but also aids thermoregulation by providing effective insulation and reducing the amount of heat lost when calves are lying down (Lorenz et al, 2011). The adequacy of pen bedding can be readily assessed using nesting scores developed at the University of Madison-Wisconsin (Van Os, 2022) (Table 2) – low scores have been associated with increased BRD prevalence (Lago et al, 2006).
Nesting score | Description |
---|---|
1 | When lying down, the entirety of the calf's hindlimbs are visible |
2 | When lying down, the calf's hindlimbs are partially visible with the metatarsi/metacarpi buried into the bedding |
3 | When lying down, the entirety of the calf's hindlimbs are buried into the bedding and are not visible |
Calf jackets may also be used to keep calves warm and dry, but studies have not demonstrated a clear health or production benefit (Earley et al, 2004; Scoley et al, 2019). Additionally, hygiene concerns have been highlighted by some authors (Robertson, 2020). Calf jackets have previously been thoroughly reviewed in this journal and readers are referred to that article for more detailed discussion (Robertson, 2020).
Calves housed individually are often considered to be at reduced risk of infectious diseases due to limited contact with other (potentially infectious) calves and accordingly, individual housing systems such as hutches have been recommended for prevention of BRD (Gordon and Plummer, 2010). Whilst individual housing is a popular choice with producers (Marcé et al, 2010), this type of housing is a consumer concern (Perttu et al, 2020) and is recognised to have negative effects on calf development (Costa et al, 2016). Furthermore, the perceived health benefits of individual housing are not well described, with studies reporting conflicting results. For example, one study found that disease incidence was higher in individually housed calves (Mahendran et al, 2023), whereas, a different study found that individual housing was associated with lower odds of developing BRD and diarrhoea (Curtis et al, 2016). Several studies have investigated pair housing of calves, consistently finding that the social benefits of group housing are maintained without negative impacts on health (Cuba et al, 2002; Whalin et al, 2018; Mahendran et al, 2021; Liu et al, 2020). Therefore, it is possible that pair housing of calves may offer the ideal compromise between limiting disease spread and facilitating social development.
Ventilation is an important consideration in prevention of BRD. A well-ventilated shed will deliver a regular supply of fresh air without being draughty, whereas poorly ventilated housing leads to high humidity and a build-up of high concentrations of pathogens and respiratory irritants such as toxic gases and dust (Figure 3).
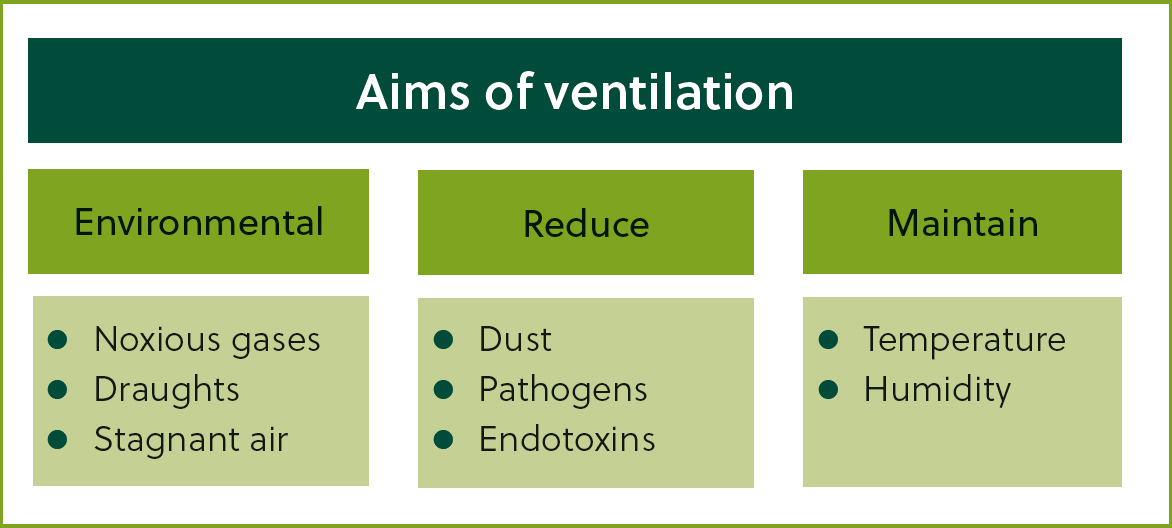
Sheds may be naturally or mechanically ventilated; mechanical ventilation can be used to supplement natural ventilation or might be the primary source of ventilation (Nordlund and Halbach, 2019). Although natural ventilation works well for housing of older youngstock and adult cattle, effective natural ventilation can be difficult to achieve in calf sheds because pre-ruminant calves do not generate enough heat to create a stack effect; therefore, supplemental mechanical ventilation can be beneficial in young calf housing. Calf shed design including ventilation has been well reviewed elsewhere (Nordlund et al, 2019; Sherwin, 2022; Robertson, 2020).
Effective biosecurity is a crucial aspect of disease prevention and has an important role in preventing BRD, not just for limiting incursion or spread of BRD pathogens but also for avoiding introduction of pathogens that might predispose to BRD such as bovine viral diarrhoea virus (BVDv). In Norway, a recent initiative has been implemented to attempt to prevent and eliminate BRD from cattle herds using biosecurity measures (Stokstad et al, 2020). Maintaining a closed herd is optimal for minimising introduction of disease (Mee et al, 2012), but this is not always possible and if purchasing of calves is needed, biosecurity strategies should be adopted to reduce the risk of introducing disease (Box 1).
Some suggested biosecurity strategies to reduce risks associated with purchasing calves (Stokstad et al, 2020; Mee et al, 2012)
Biosecurity within the herd should be focused on limiting spread of BRD pathogens from infected animals to healthy animals, especially those who may be immunocompromised such as newborn calves and postpartum cows. Sick calves should be isolated from healthy calves, preferably in a separate housing area dedicated to this purpose. Sick calves are ideally handled after healthy calves and disinfectant and appropriate protective clothing should be provided so that pathogens are not spread by movement of staff through the farm.
Stress
Stress is thought to predispose to BRD infections through a combination of immunosuppression and alteration of the respiratory mucosa (Taylor et al, 2010). Transport stress is widely accepted to be a risk factor for BRD and has given rise to the term ‘shipping fever’ to describe BRD outbreaks occurring shortly after transport. Studies investigating associations between BRD and transport stress are often performed on large feedlots, which may have limited relevance to UK systems. However, a large case-control study investigating BRD risk factors in (non-feedlot) Irish herds found increasing numbers of movements was associated with increased odds of developing BRD in calves aged between one and six months (Murray et al, 2016).
Stressful management events such as weaning and painful husbandry procedures may also increase the risk of BRD infection, but results of studies are conflicting and the complexity and multifactorial nature of BRD makes it difficult to be able to accurately determine the importance of these types of stressors (Gorden and Plummer, 2010; Taylor et al, 2010). Nevertheless, it is prudent to minimise stress wherever possible for welfare reasons as well as for any potential health benefits.
Control and management of BRD outbreaks
Investigations of BRD outbreaks should include taking a thorough history and clinical examination of affected animals. Several clinical scoring systems have been developed to aid diagnosis of BRD (McGuirk and Peek, 2014; Jaureguiberry et al, 2023; Møller et al, 2024); one well-known example is the Madison-Wisconsin calf respiratory scoring chart (University of Madison-Wisconsin, 2024). Different scoring systems demonstrate high agreement with each other (Love et al, 2014) but their sensitivity can be low (Donlon et al, 2024) and the accuracy of BRD detection using these systems is subject to debate (Buczinski et al, 2015). Thoracic ultrasonography (TUS) has been proposed as an alternative method for detecting BRD that is more specific for lower respiratory tract disease. A further advantage of TUS is the ability to detect areas of lung consolidation in clinically unaffected calves which may enable earlier detection and treatment of disease (Porter et al, 2021; Cuevas-Gómez, 2021). A recent review concluded that using clinical scoring systems and TUS together may lead to more accurate BRD detection than using either method alone (Reynolds and Brennan, 2021), a hypothesis supported by Cuevas-Gómez et al (2021), but more research is warranted to explore this further.
Obtaining samples from the lower respiratory tract may be useful in some cases, particularly in cases where identification of specific pathogens has value (such as if farmers want to implement a targeted vaccination programme). However, interpretation of results can be complicated by the presence of co-infection and some BRD pathogens may be difficult to isolate, potentially leading to false negative results. Similarly, whilst postmortem examination can be valuable for obtaining a gross diagnosis of BRD, pathogens isolated from calves who have died may not be representative of the rest of the group, especially if the deceased calf was chronically affected. As such, caution needs to be taken when making treatment decisions based on results of postmortem examination.
Antibiotic therapy is the mainstay of BRD treatment and achieving good responses to initial treatment is key to achieving positive longer-term outcomes (Lorenz et al, 2011). Therefore, it is important that antibiotics are selected and used appropriately. Nonsteroidal anti-inflammatory drugs (NSAIDs) are widely used in the treatment of BRD due to their anti-pyretic and antiinflammatory effects. Improvements in lung lesions, production parameters and clinical signs have all been reported in calves and youngstock treated with NSAIDs in addition to antibiotic therapy (Bednarek et al, 2003Lockwood et al, 2003; Masebo et al, 2024) and inclusion of NSAIDs in BRD treatment protocols is recommended. The use of NSAID treatment without accompanying antibiotic treatment has been explored, but the benefits of this approach are as yet unclear. Mahendran et al (2017) found that clinical signs in a quarter of calves treated with only flunixin resolved without the need for antibiotic therapy but calves in the flunixinonly treatment group were also five times more likely have pyrexia persisting at 72 hours after treatment than calves in the antibioticonly treatment group (Mahendran et al, 2017). These results suggest that for many calves, initial NSAID treatment alone may delay administration of antibiotic treatment but for some calves there may be benefits to this approach. If NSAID treatment is used as an initial treatment without antibiotic therapy, close monitoring of calves (including regular temperature measurement) is warranted so that calves who do not respond can be identified without delay.
Conclusion
Bovine respiratory disease is a complex, multifactorial condition that has wide-ranging negative economic and welfare impacts. Prevention strategies for BRD need to address the joint aims of a) enhancing disease resistance at herd and individual level together and b) reducing exposure to BRD pathogens. Where BRD outbreaks occur, affected calves should be detected and treated promptly to maximise the likelihood of positive outcomes.