Trace elements impact the health and productivity of UK sheep flocks when supply is not optimal. The consequences of suboptimal supply can result in various clinical presentations (Table 1). However, in some instances, unproven trace element deficiencies and their correction have been lauded as a panacea for all manner of conditions (Hinde, 2020). Therefore, it is important to gather sufficient evidence to support a presumptive diagnosis and justify laboratory test expenditure. These tests are often not as diagnostically defini- tive as we would like (Clark and Wright, 2005) and should be interpreted in conjunction with background evidence. In addition, a better understanding of the factors contributing to each particular presentation of deficiency may improve targeted nutritional management.
Table 1. Clinical presentations that could be associated with trace element deficiencies in sheep and some considered differential diagnoses
Stage of production | Problem | Indicator | Trace element | Major differential diagnoses |
---|---|---|---|---|
Breeding/pregnancy | High return rate | Raddle markingsOngoing ram activity >35 days | ZincSeleniumIodine | Poor ram fertility, low ram:ewe ratio, low ewe ovulation rate, poor breeding conditions |
Low scanning percentage | Ultrasound pregnancy scanning results | ZincCobaltSeleniumIodine | Above plus: undernutrition, infectious disease, parasites, prolonged stress, medications | |
High barren rate at lambing | Empty or unproductive ewes | ZincCobaltSeleniumIodine | Above plus: infectious abortion, severe stress, toxins, severe nutritional restriction | |
Extended lambing | <60% of lambs born in the first 17 days | SeleniumIodineCobaltZinc | Poor ram fertility, low ram:ewe ratio, low ewe ovulation rate (low body condition etc), poor breeding conditions | |
Peri-natal period | Ewe colostrum/milk quality | Poor lamb survivalPoor lamb growth rates | Cobalt | Nutritional insufficiency, low ewe BCS, parity, maternal disease |
Stillbirths Weak lambs | StillbirthsPoor lamb survivalWeak lambs | IodineSeleniumCobaltCopper | Toxoplasma, Campylobacter, enzootic abortion of sheep, border disease virus, poor ewe nutrition and BCS, maternal disease, toxins, genetic predisposition | |
Swayback | Clinical signs | Copper | Congenital form
|
|
Growing lambs | Sudden death | Sudden deathHigh mortality rates | Selenium | Clostridial disease, Pasteurellosis, misadventure |
Reduced mobility | Not keeping up with the damIncreased lying time | SeleniumCopper | Spinal abscess, trauma, joint ill, vertebral body osteomyelitis, pneumonia | |
Ill thrift | Reduced 8-week lamb weightsAnorexia | CobaltSeleniumCopper | Parasitic gastroenteritis, coccidiosis, nematodirosis, liver fluke (autumn/winter), poor nutrition, poor milk production of ewes, lameness, chronic suppurative disease | |
Miscellaneous | Adult ill thrift | Ill thrift, poor wool | Cobalt (severe)Selenium (severe) Iodine | Poor nutrition, poor dentition, iceberg diseases (MV, Johnes, OPA), liver fluke, sheep scab, chronic pneumonia |
BCS, body condition score; MV, Maedi Visna; OPA, ovine pulmonary adenocarcinoma
Gathering such evidence requires thorough history-taking, which will be the focus of this article. This history-taking goes beyond the scope of individuals or groups of animals to encompass a holistic understanding of farm management and geographical factors. These factors may influence the likelihood that a clinical scenario results from a trace element imbalance, thereby moving trace element imbalance up or down a differential diagnosis list.
Ascertaining if a clinical scenario is the result of an underlying trace element imbalance requires investigating the provision of that element in the previous and current diet. Therefore, obtaining a thorough dietary history for the affected group of animals over 3, or even 6, months is critical for assessing poor supplementation. All dietary input over the relevant months should be accounted for during an investigation. This includes:
- Conserved forage
- Pastures grazed (including all fields grazed during the period of interest, also brassicas, fodder beet)
- Concentrate feed
- Mineral and feed supplements (eg buckets or blocks)
- Anthelmintic drenches containing trace elements (eg selenium and cobalt).
In addition, an assessment of access to and intake of the diet on offer should be made, as feed intakes may vary within a group if feed space or palatability are poor. Ideally, samples from each dietary constituent would be retained and analysed for trace element concentration or frozen at the time of feeding in case analysis is required at a later date.
Dietary composition and presentation are also important as they influence both feed intake and the ruminal environment. Changes to this environment (eg pH variation) may affect trace-element bioavailability; for example, a low rumen pH can increase the antagonistic binding of copper (Cu), reducing its bioavailability (Gould and Kendall, 2011).
In the authors' experience, most clinical scenarios related to trace element deficiencies are associated with animals at pasture. Therefore, this article will focus on pasture-associated factors impacting trace element provision and availability.
Clinical history
When presented with a clinical scenario, a standard clinical history needs to be taken. This should include the following:
- The clinical signs observed
- How do these differ from the farmer's expectations
- Their duration and progression
- The proportion of animals affected per group
- The age of affected animals
- If similar signs have been seen before
- If treatment was attempted and what, if any, was the response
- Have any other group issues been noted in the flock in recent years?
Signs associated with trace element deficiencies can affect various areas of flock performance. Assessing recent flock performance compared with previous years' or reasonable targets can highlight areas of concern. The investigation could include (but should not be limited to):
- Scanning results – the percentage of ewes that were barren, the percentage of ewes carrying single, twin or triplet lambs
- Lamb vigour at birth
- Lamb weaning rate
- Lamb growth rates
- Lamb age at finishing
- Fleece quality.
Taking the long view of a farm's clinical history can strengthen or weaken the suspicion that TEs are involved in a clinical syndrome.
For example, there may be a history of poor autumn lamb growth, which increases our suspicions of trace element involvement when presented with a fertility problem. Such co-morbidities can often move trace element deficiency up the differential diagnosis list. Other areas of sheep health to assess that may influence flock performance include overall nutrition (energy, protein, macrominerals), ewe body condition scores (absolute scores and fluctuations), nematodes, liver fluke, sheep scab and the presence or absence of iceberg diseases.
Local knowledge
The possibility of a TE-related problem occurring on a farm can be predicted to some degree from local history. As a new graduate, or when moving to a practice in a new location, it can be helpful to call upon the experience of local vets, farmers or surveillance service officers (APHA and SRUC). These sources may know if farmers in a particular valley have previously struggled to finish lambs off pasture or if goitres were once a typical post-mortem finding in lambs from another area. If specific local knowledge is unavailable, resources such as the UK Soil Observatory (Figure 1) provide maps that give a broad overview of UK soil trace element concentrations at a granularity of 1 km2.
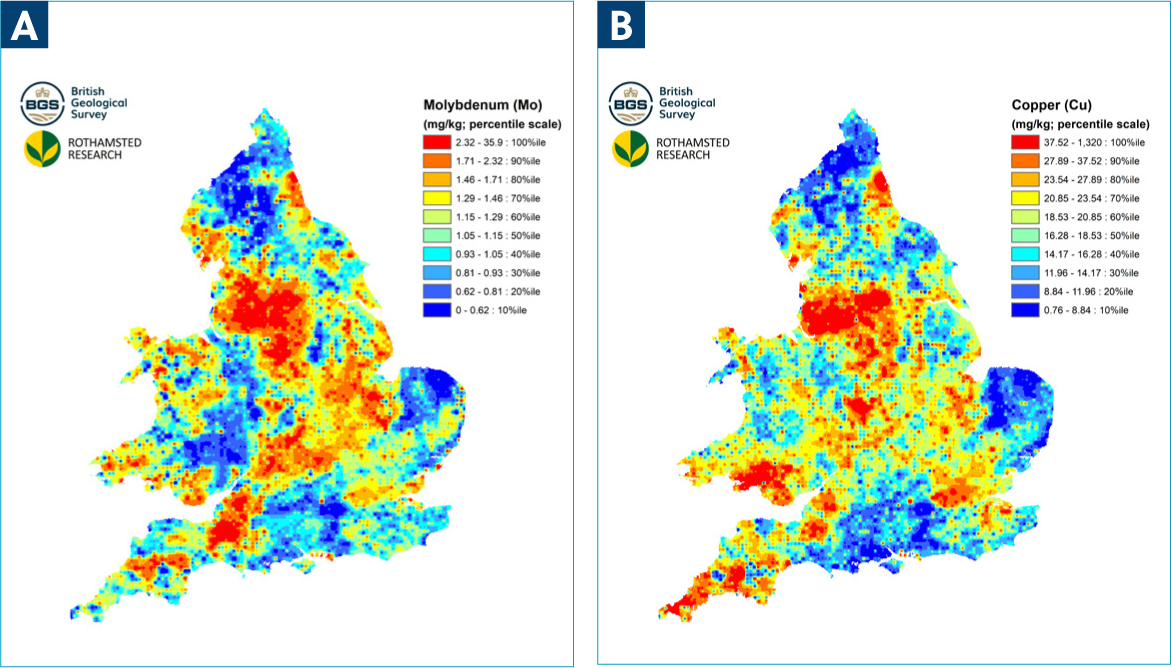
Even routine management practices, which are inherited or appear to be habitual, could be pertinent. Information concerning these is not always volunteered during a standard history discussion with clients, and therefore requires intimate questioning or knowledge of the farm's practices. For example, lambs may never be grazed on specific fields after weaning, or other areas are avoided for ewes at flushing and breeding time. This suggests historical knowledge of a geographically associated clinical presentation, which may have been associated with trace element deficiency.
It is important to remember that these factors only indicate a potential for trace element involvement and do not provide sufficient evidence to make a definitive diagnosis.
Soil type
In addition to the collective historical knowledge of syndromes associated with trace element deficiency, quantitative and qualitative aspects of an area, farm or particular field can indicate risk. Soil type can be used to estimate which trace element may be over- or under-supplied and the availability of those trace elements for uptake by plants in a particular pasture. Soil type can vary between fields across a farm, and farmers may be able to advise on which soil types are present. If not, maps from the British Geological Survey provide a rough indication of the soil type in an area (Figure 2). Table 2 provides a simplified summary of the relative risk associated with the major soil types. However, other soil characteristics can influence trace element availability; for example, high organic matter content can limit Cu availability.
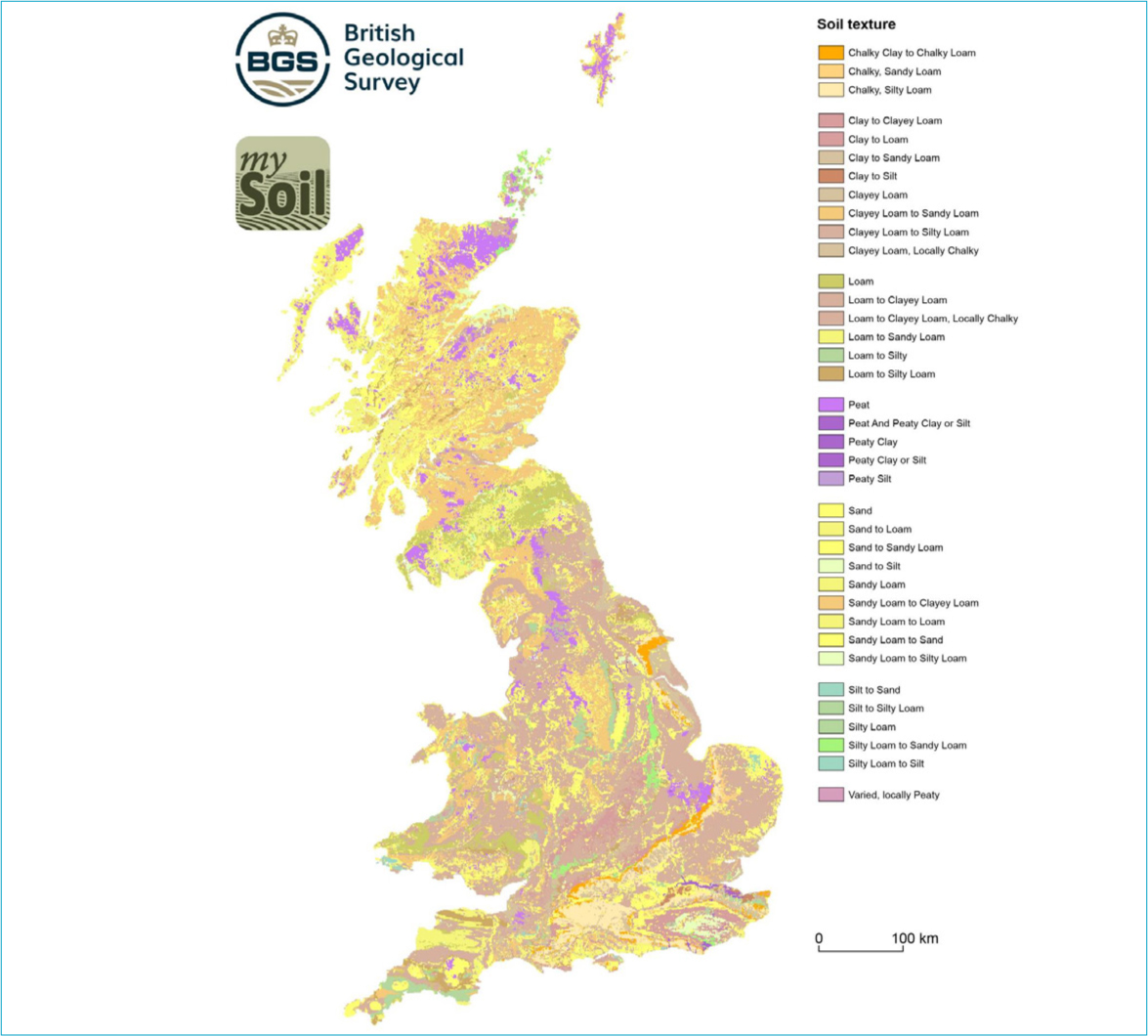
Table 2. Relative risk of deficiency as defined by likely low soil content and plant availability
Mineral | Calcareous | Clay | Peat | Sand |
---|---|---|---|---|
Cobalt | Variable soils implicated in cases of deficiencies | |||
Copper | High | Moderate | Low | Low |
Iodine | Moderate-high | Moderate | High | Moderate |
Selenium | Low-moderate | Moderate | High | Low-moderate |
Zinc | - | - | - | High |
Soil pH
Soil pH affects the uptake of trace elements by plants. As with soil type, farmers may know the pH of their soils; if not, the UK Soil Observatory (2023) provides an interactive map on which this information can be found. Soil pH affects the plant's ability to absorb TEs, and different pHs benefit or hinder the uptake of different elements. In Figure 3 (Suttle, 2010), we see that acidic soils benefit cobalt (Co), manganese (Mn) and zinc (Zn) uptake, and as soil pH increases, plant uptake of these elements decreases. Soil pH does not significantly affect plant Cu uptake; however, molybdenum (Mo) uptake increases as soils become more alkaline. Molybdenum is a Cu antagonist; therefore, the improved plant Mo availability seen in alkaline soils can increase Mo consumption, reducing animal Cu availability, even if sufficient Cu is being consumed. The effect of soil pH on iodine (I) uptake varies depending on the form of I in the soil. Iodide uptake by plants is improved in acidic soils, while iodate uptake is improved in alkaline soils. Kao et al (2020) has more information on the factors that affect soil and plant concentrations of trace elements.
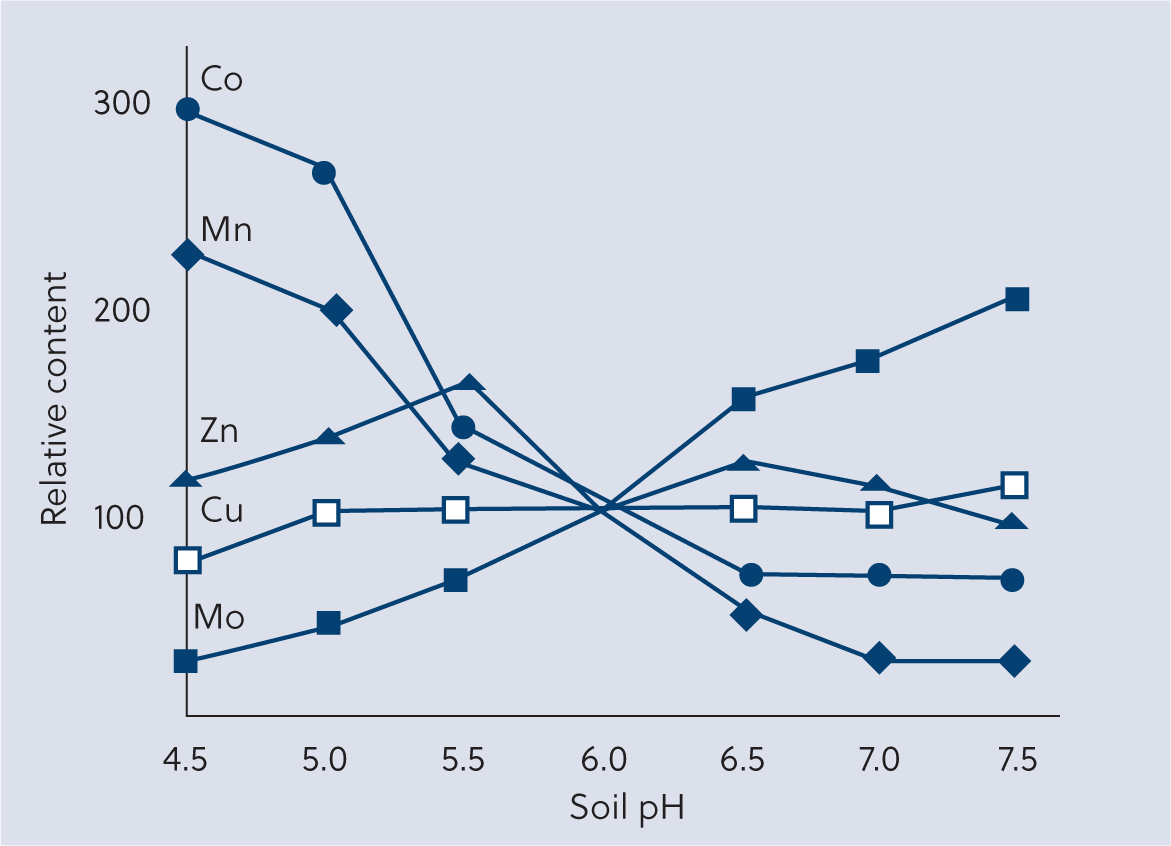
Soil pH can differ within a field; therefore, plant trace element concentrations can vary considerably in different sections, even in monoculture pastures.
Pasture
Plant species vary in trace element concentrations. Generally, herbaceous plants (eg chicory and sainfoin) have higher trace element concentrations than grass-type plants, whereas leguminous plants, like clover, can have higher Co, Cu and Mo (Burridge et al, 1983; Kincaid et al, 1986) but lower I concentrations when compared to grass. Certain plant species, like forage brassicas, are fed for a limited time, primarily as winter forage. Most brassicas contain low trace element levels and anti-nutritional constituents (eg high sulphur (S) levels and goitrogenic compounds), which limit trace element absorption and utilisation (see the antagonists and interactions section).
Not only do trace element concentrations differ by plant species, but they also differ by stage of plant maturity and plant section (root, stem, leaf, flower; Table 3). Co, Cu and Zn tend to be concentrated in the leaf and flower heads rather than in the stem (Blom, 1934; Fleming, 1963; Davey and Mitchell, 1968), so the concentration of these trace elements in the plant reduces as the stem-to-leaf ratio increases with increasing maturity. Consequently, trace element concentration decreases as the season progresses from spring to late summer (Alderman and Jones, 1967; Fleming and Murphy, 1968; Whitehead and Jones, 1969; Fleming, 1970; Minson, 1991).
Table 3. Comparative trace element concentrations in different plant sections and at various stages of plant maturity
Plant parts | ||
---|---|---|
Cobalt | Leaves > flowerheads > stem | Davey and Mitchell (1968); Fleming and Murphy (1968) |
Copper | Leaves > stems | Davey and Mitchell (1968) |
Iodine | Leaves > stems | Blom (1934) |
Zinc | Flowering heads > leaves > stem | Fleming (1963) |
Maturity | ||
Cobalt | Content reduces as leaf content reduces but may be inconsistent in clover | Fleming and Murphy (1968); Fleming (1970); Whitehead and Jones (1969) |
Copper | Content reduces as leaf content reduces and stem matures | Minson (1991) |
Iodine | Concentration decreases over the growing season | Alderman and Jones (1967) |
Selenium | Potential seasonal variation with lower values in late summer | Gissel-Nielsen (1975) |
Zinc | Inconsistent reduction with maturity | Gladstones and Loneragan (1967); Whitehead and Jones (1969) |
This within and between plant variability (Figure 4) makes measuring and interpreting the trace element content of pasture challenging. Previous pasture testing may no longer represent the current diet fed, and current pasture testing may no longer reflect the diet fed when the dietary insult occurred. Samples for analysis should, therefore, be taken from several points within a field and at various times throughout the year to build a robust picture of the evolving pasture trace element content. Nevertheless, selective grazing will further impact trace element consumption levels, necessitating that pasture testing is interpreted in conjunction with animal-based evidence.
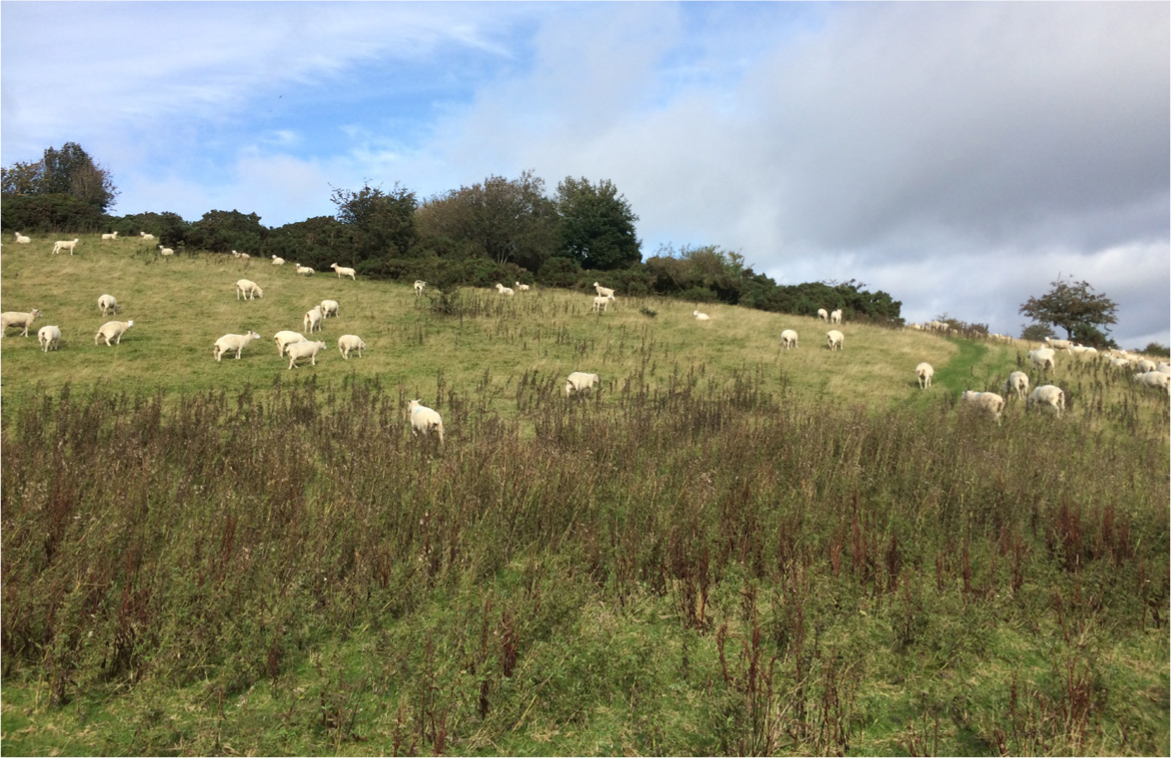
Weather
Recent weather and longer-term climactic conditions will influence pasture trace element contents as both soil concentrations and plant uptake will be affected (Spears, 2003). In high-plantstress situations, like droughts, heat waves or cold snaps, trace element uptake will decline as a result of low root activity. Some weather events can result in a beneficial change to pasture trace element status. For instance, selenium (Se) uptake by plants may increase in dry weather. In contrast, Co, Mn and Mo (and, to a degree, I) uptake can increase in wet or waterlogged conditions (Burridge et al, 1983). However, extreme waterlogging can lead to the leaching of trace elements from pastures, especially I (Figure 5).
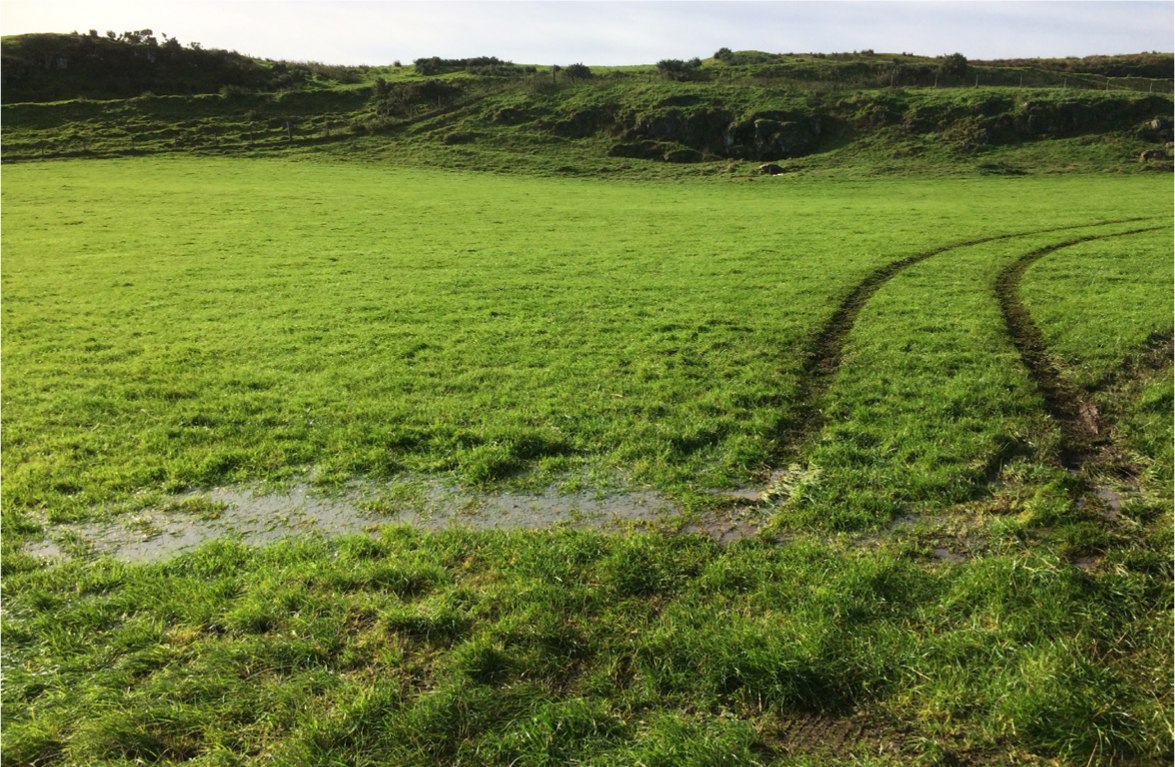
Water may also be a source of trace elements and can be variable when natural sources or bore-hole water is supplied.
Element antagonists and interactions
As previously mentioned, certain trace elements and biologically active compounds can influence the absorption and metabolism of other trace elements by plants and animals (Table 4; Table 5).
Table 4. Antagonists of the commonly supplemented trace elements
Element | Antagonist | Site |
---|---|---|
Cobalt | ↑ Manganese | Reduces plant absorption |
Copper | ↑ Iron, molybdenum, sulphur↑ Zinc | Reduces animal absorption/retention |
Iodine | Goitrogens (↑ Calcium) | Inhibits utilisation |
Selenium | ↑ Phosphorus, sulphate↑ Molybdenum, sulphur, (calcium) | Reduces plant absorptionReduces animal absorption |
Table 5. Conditions that favour or inhibit trace element availability for grazing animals
Factor | Cobalt | Copper | Iodine | Selenium | Zinc |
---|---|---|---|---|---|
Soil type | Iron-rich, alkaline, manganese-rich ↓ | Calcareous, clay, iron, manganese, molybdenum, sulphur-rich↓ | Inland, calcareous ↓ | Acidic, peat ↓ | |
Fertilisers | Lime: ↓ | Nitrogen, sulphur: ↓ (Lime: ↑ molybdenum) | Nitrogen: ↓ (Nitrogen + sulphur: ↓ goitrogens) | Sulphur: ↓ Lime: ↑ | ↑ |
Pasture type | Leguminous plants ↑ | Leguminous plants ↑ (↑molybdenum) | Leguminous plants ↓ | Leafy brassicas ↑ | Very variable |
Rainfall/drainage | Low rainfall / well drained ↓ | Excessive rainfall ↓ (↑molybdenum) | Excessive rainfall ↓ | High rainfall ↓ | |
Season | ↓ later in summer | ↓ later in summer | ↓ later in summer | ↓ later in summer |
The effects of Mo and S on Cu are well documented (Suttle, 1974; 1991; Mason et al, 1982; Price et al, 1987; Suttle, 1991). Mo and sulphides combine in the rumen to form thiomolybdates, which form insoluble complexes with Cu, making the Cu unavailable for absorption by the ruminant. Thiomolybdates may also be absorbed into the bloodstream, causing:
- Cu release from the liver into the bile
- An increase in Cu binding to albumin, which reduces Cu transport
- Scavenging of Cu from metalloenzymes.
Sulphur can reduce Cu bioavailability independently of Mo by forming insoluble Cu-sulphide in the gastrointestinal tract. The antagonistic effect of Fe on Cu is less well described but occurs only in the presence of S and only in ruminants.
Goitrogenic compounds are the main antagonists of I. These goitrogens are mainly found in brassicas, with levels varying depending on the species, growing conditions and growth stage. Modern brassicas contain lower concentrations of goitrogens than traditional cultivars. Goitrogens can act in two ways (Suttle and Jones, 2007): as thyroperoxidase inhibitors preventing the incorporation of I onto thyroglobulin when producing thyroid hormones (T3 and T4); and as an iodothyronine deiodinase inhibitor preventing the conversion of T4 to T3. Identifying the type of goitrogen involved can help determine the correct treatment. This may be achived by contacting the seed supplier for the variety of plant under suspicion. Supplementation with additional I will successfully counter thyroperoxidase inhibitors, whereas animals should be removed from pastures containing excessive iodothyronine deiodinase inhibitors (Suttle, 2010). Excess Ca in the diet has also been reported to interfere with the I status of animals.
High levels of phosphorus and S in soil can inhibit Se uptake by plants, as they are absorbed through similar pathways, thereby in competition. High dietary S can also reduce Se uptake in the animal as it is chemically similar to Se and therefore competes for receptors (Gupta and Gupta, 2017).
Pasture management
Some pasture management practices can increase the risk of trace element deficiency. Therefore, knowing how pastures were managed could guide a clinical investigation (Table 6). Poor pasture management or adverse weather conditions can lead to overgrazing, poaching and subsequent soil ingestion. Soil ingestion can be beneficial by increasing Cu, Co, I, Se and Zn uptake. However, it can also be detrimental by increasing Fe and Mo uptake, thus reducing Cu absorption (Givens et al, 2000).
Table 6. Nutrition history questions to guide trace element deficiency investigations
What forage, concentrates and supplements have the animals been fed in the last 3–6 months? How is each part of the diet fed? Assess the accessibility of each element to the whole group. |
Is there a history of trace element deficiencies
|
For any relevant fields:
|
What time of year was the pasture being grazed? |
What was the weather like during grazing? |
Fertilisers change the chemical composition of topsoil and plant growth. The application of a nitrogen fertiliser increases plant growth, which can reduce the concentrations of some TEs in the plant, particularly Cu and I (Hopkins et al, 1994). Magnesium, Na and Zn concentrations have been shown to increase when nitrogen fertiliser is administered, although this is inconsistent.
As noted previously, soil pH affects trace element uptake by plants. High S fertilisers can acidify soil, resulting in reduced Se uptake (Murphy and Quirke, 1997). Conversely, lime increases soil pH, reducing Co (Archer, 1970), Mn and Zn (Curtin and Smillie, 1986; Gray et al, 2006) and increasing Mo uptake by plants, which interferes with Cu availability (Stewart, 1951).
Other management practices, such as harvesting grass for silage or hay, can reduce Zn concentrations in re-grown herbage. Each successive cut results in lower Zn concentrations, which cannot be remedied by applying Zn-rich fertilisers (Underwood and Suttle, 1999).
Both grass and soil mineral analysis are common tools used by farmers when investigating poor crop/grass yields or when planning a fertiliser protocol with an agronomist. However, they can also be used to indicate when pastures pose a risk of clinical trace element deficiency to livestock. As with animal-based testing, understanding what, when, and how samples were tested is essential for appropriate interpretation.
Grass should be collected at regular intervals in a zigzag or ‘Z’ pattern across a field at several time points throughout the grazing season. Handfuls of grass should be cut with scissors near the base of the stem, placed in a bag, mixed, and immediately refrigerated. Tearing grass from the ground will likely result in soil contamination and erroneous test results. Samples should be sent for analysis as promptly as possible.
Soil testing is most useful when soils within the root growth area are sampled and tested for plant-available nutrients, as the total nutrient content does not guarantee plant sufficiency (Horneck et al, 2011). Soils from permanent pastures can be tested every 2–3 years, ensuring that tests are performed at the same time of year and in roughly the same area to allow for consistency. Unless significant work has been done to alter the nutrient composition of soils between tests, large variations are likely to indicate inconsistent sampling procedures; therefore, pastures should be re-sampled. Nutrient-specific information on soil test interpretation can be found on Oregon State University's extension service webpage (https://catalog.extension.oregonstate.edu/).
Conclusions
Sheep can be clinically affected by inadequate (or excess) trace element provision. Many factors can influence the availability of these elements. In order to determine whether trace elements are implicated in a clinical presentation, a thorough investigation must be undertaken. This includes a detailed history of the clinical presentation, nutrition and grazing, including the permanent and temporary characteristics of the pasture grazed (Tables 5 and 6). Many of the clinical trace elements conditions encountered have numerous common differential diagnoses. These must be ruled out in order to confirm trace element deficiency as the sole cause of disease.
KEY POINTS
- Trace elements are important for the health, welfare and productivity of sheep, but can be overused.
- Recent flock production can help determine whether trace elements are culpable in a clinical scenario.
- Understand what affected animals have been fed in the previous 3–6 months.
- Use local knowledge and recent weather patterns.
- Interpret soil and pasture trace element results carefully alongside animal information.