Swine influenza A virus (SIV) can have a large economic impact on the pig industry, and the zoonotic threat also makes it a concern for public health. A study has shown that 56% of UK farms were positive for influenza, based on 146 samples from nine farms. The same study also showed Ireland to have a number of positive farms for pandemic SIV, H1pdmN1pdm (Henritzi, 2019). Biosecurity and hygiene protocols should be implemented on farm to reduce environmental contamination and therefore the risk of pathogen spread. Understanding of diagnostic testing is extremely important in implementing the correct vaccination protocol on farm and the various methods of testing and the vaccination options will be discussed in this article.
The pathogen
Swine influenza is caused by influenza A virus which is an orthomyxovirus 80–120 nm in diameter, comprising eight pieces of segmented RNA coding for 12 proteins, including haemagglutinin (HA), neuraminidase (NA) and matrix 2 proteins which form part of the virus envelope (Figure 1). The virus is classified by type A, B, C or D, which is based on the matrix and the nucleoprotein. The subtypes are then defined by the haemagglutinin (HA) and neuraminidase (NA) proteins (Sandbulte et al, 2015). New subtypes can develop by antigenic shift, antigenic drift or reassortment. Three reassortment porcine lineages circulate in swine in Europe, including H1avN1av, H1huN2 and H3N2. However, the human pandemic H1N1/2009 virus has established a fourth lineage in swine, H1pdmN1pdm (Henritzi et al, 2016).
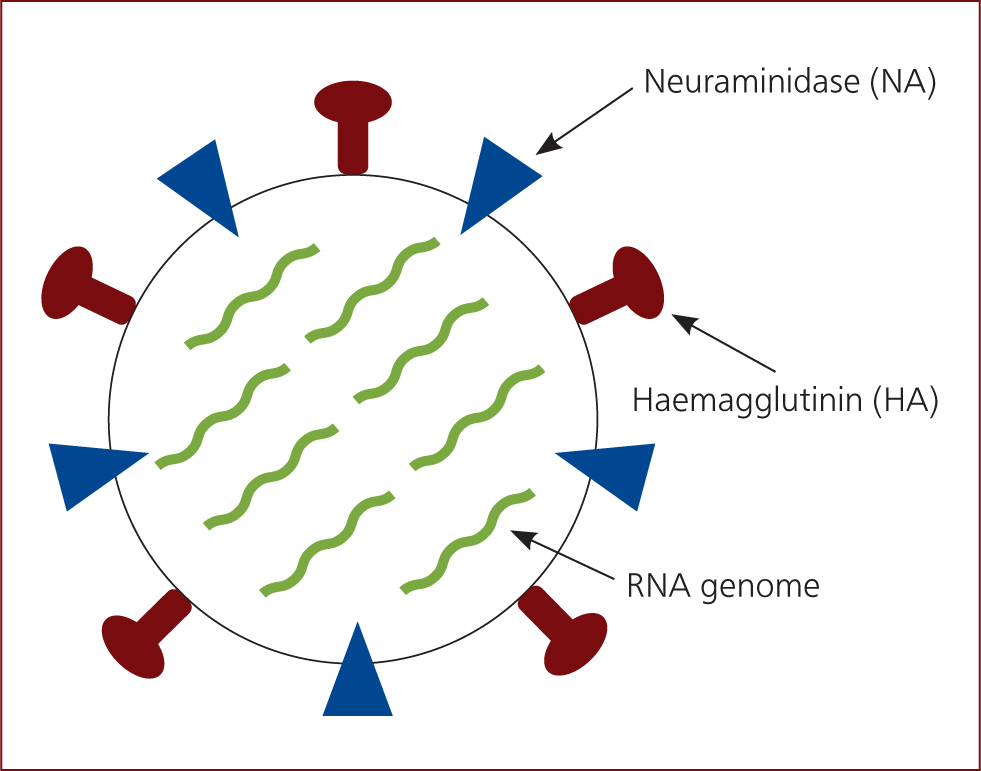
Antigenic shift occurs where there is a change in the host, for example, H1N1 in poultry being directly transmitted to pigs. This results in the development of new subtypes via double or multiple infections of a cell with two different subtypes or genetic lines. This can lead to the exchange and free combinability of the eight gene segments. Exchange of gene segments between two influenza viruses that infect the same animal is known as reassortment. New viruses are produced which have different combinations of proteins on their surface, with potential new HA or NA proteins, which results in these new subtypes being able to invade the immune system as the population does not have antibodies to protect them. Antigenic drift is a more gradual process where accumulated point mutations occur to the HA and NA proteins, causing a variation within a subtype. This can also result in the virus being able to evade the immune system, but the process is much slower than with antigenic shift (Sandbulte et al, 2015).
The natural host of all influenza A viruses is wild birds, however the presence of specific receptors in other animals allows cross-species transmission. These receptors include α2,3 which are commonly found in avian and α2,6 which are commonly found in humans. Swine have both α2,3 and α2,6 receptors meaning they can be infected by both avian- and human-origin influenza-A viruses. Therefore, swine are referred to as the ‘mixing vessel’ for the influenza virus, because they act as an intermediate host (Figure 2) (Ma et al, 2009).
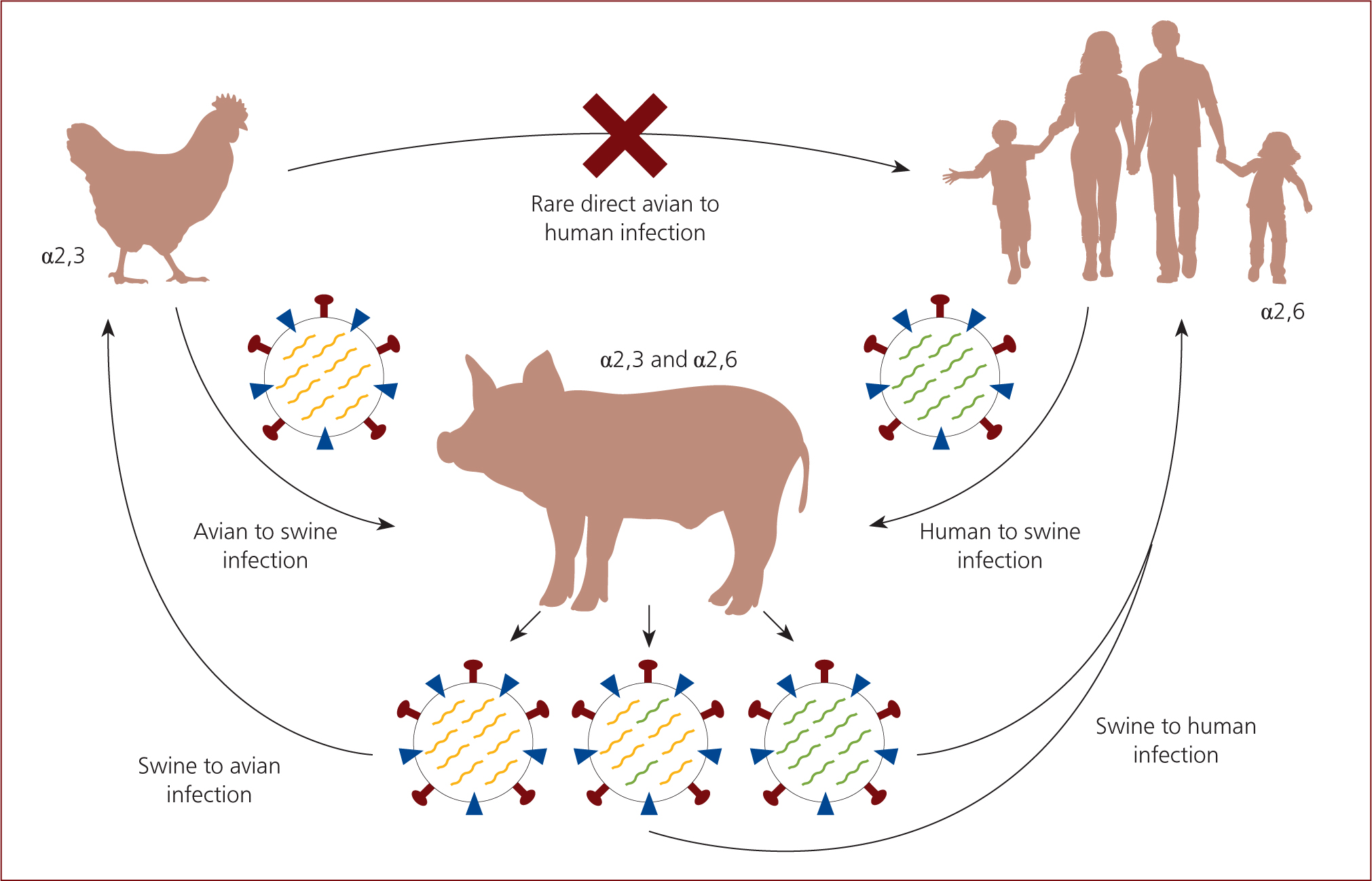
Pathogenesis
The virus enters the respiratory tract and multiplies rapidly in cells of the bronchial epithelium. It is the HA protein that is responsible for the binding of the virus to the host cells (Zhu et al, 2013), by interacting with the sialic acids linked to proteins on the host cell. Once this binding is complete, the virus can enter the host cell (Samji, 2009). The NA protein then has a role in ensuring that the infected cell can release more virus which results in efficient spread to uninfected cells (Ma and Richt, 2010). This occurs until around 24 hours post infection where most cells are infected and exudate is present. Infection is usually mostly gone by day 9. Small bronchi become blocked by exudate, hyperplasia of the bronchial epithelium and necrosis of alveoli occur, all contributing to the clinical signs observed in infected animals. Macroscopic lung lesions may be present at necropsy characterised by multi-focal purplish-red consolidated areas on lung lobes, primarily in the cranioventral areas (Sreta et al, 2009) (Figure 3). Influenza cases can be made worse by concurrent infection with Actinobacillus pleuropnuemoniae, Glaeserella parasuis and Pasturella multocida (Taylor, 1995).
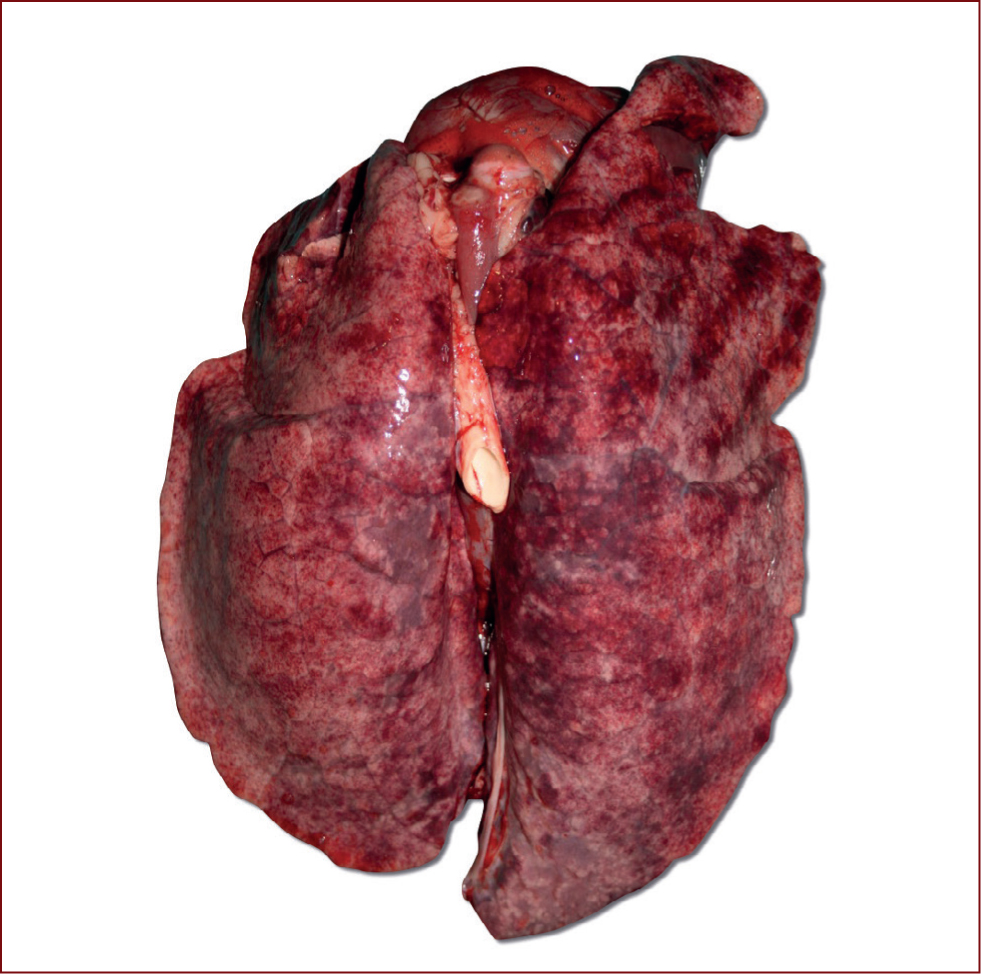
Epidemiology
The virus can spread between pigs via various routes including aerosol, droplet infection and direct contact snout to snout. However, the virus does not persist for long in the environment and is sensitive to detergents and disinfectants as well as heat and drying. The disease is more common in cool conditions and therefore is observed more in the winter months. Maternal immunity may be demonstrated for up to 4 months, however it may not prevent infection and can interfere with the development of active immunity (Taylor, 1995).
Clinical signs
The incubation period for SIV is 1–2 days and usually nearly all susceptible animals are affected. Clinical signs include increased abortions and return to oestrus in sows. Respiratory signs include coughing, sneezing and nasal secretions which may be accompanied by fever, lethargy and lack of appetite therefore resulting in poor growth and weight loss. Between days 5–7 recovery usually occurs and mortality is low (less than 1%) (Taylor, 1995). In the classical form of the disease, all stages may be affected and cases are periodic. In the endemic form there are often no clear clinical signs, there is an increase in reproductive disorders and severe respiratory disease in piglets of around 6–7 weeks of age. Disease often associated with pandemic strains may be seen as the ‘atypical’ form and results in increased reproductive disorders including decreased number of piglets born live, increased non-viable piglets, decreased milk production of sows and increased abortions (Gumbert et al, 2020).
Coinfections
Swine influenza can form part of the porcine respiratory disease complex (PRDC) as a primary viral agent, it can be joined by a primary bacterial agent such as Mycoplasma hyopneumoniae and A. pleuropneumoniae, and also predispose to secondary bacterial infection. These coinfections have an important impact on the farms due to the effect they have on pig production profitability. Studies analysing coinfections with porcine respiratory and reproductive syndrome (PRRSV) have demonstrated a lower mean weight gain when the pigs are challenged by PRRSV and SIV in comparison to single virus infections (Van Reeth et al, 1996).
In dual infections with M. hyopneumoniae, the clinical disease, measured by coughing, was greater in the pigs in the dual group than in the separate infections (Thacker et al, 2001).
Another study evaluated the association between A. pleuropneumoniae and influenza and determined that the coinfection with SIV and A. pleuropneumoniae potentiated the severity of lung lesions caused by SIV and enhanced virus replication in the lung and nasal SIV shedding. This enhanced SIV replication contributed to a more severe clinical course of the disease as well as earlier and higher magnitude immune response compared with single infected pigs (Pomorska-Mól et al, 2017a).
In relation to G. parasuis, SIV increased the severity of lung lesions and facilitated G. parasuis replication in the lung. Furthermore, G. parasuis influenced the nasal shedding of SIV. Enhanced G. parasuis and SIV replication, together with stronger systemic and local inflammatory response contributed to more severe clinical signs (Pomorska-Mól et al, 2017b).
Diagnosis
Influenza can be hard to diagnose as infected animals only shed the virus for a short period of time and the prevalence within the batch or affected group may be low. In the case of pandemic strains this can make detecting the virus difficult. Due to this, different clinical signs in different age groups require different sampling strategies. Virus detection can be carried out using various samples including nasal swabs (Figure 4), bronchial swabs, oral fluids and serum (Sandbulte et al, 2015). If there are clear clinical signs, including high incidence, fever, dyspnoea and mortality in pigs and abortions and increased return to oestrus then nasal swabs or bronchoalveolar lavage fluid (BALF) should be taken. Nasal swab samples should be taken from pigs with high fevers and nasal discharge (it is important that the samples are taken when the clinical signs have been present for 1 or 2 days maximum, as clinical signs persisting for longer may be due to secondary bacterial infections and therefore false-negatives for SIV may be recorded), and should then be kept moist by placing into small vials or cell culture media to ensure the virus is not inactivated (Janke, 2000). These swabs can then be used for polymerase chain reaction (PCR) analysis, and if required can be used for cell culture to isolate the virus.
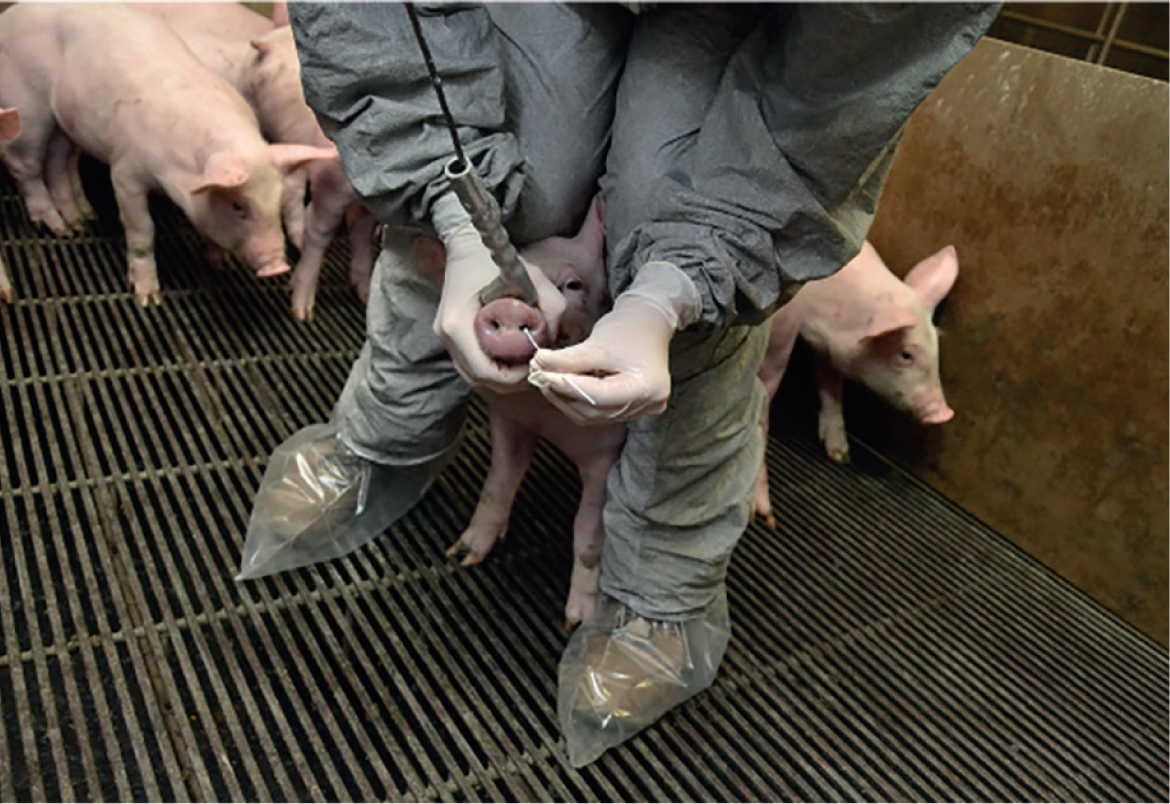
Antibodies can be detected using haemagglutination inhibition (HI) testing and enzyme-linked immunosorbent assay (ELISA). The ELISA test does not differentiate the subtypes present in the samples, but it is very sensitive to all the SIV antibodies. The HI test identifies the different subtypes (H1N1, H1N2, H3N2 and even the pandemic strains H1pdmN1 and H1pdmN2). The results and the titre concentration will depend on the similarity between the reference strain used in the lab and the field strain. The HI test is considered the gold standard and detects the circulating antibodies in the serum that bind to the virus in the test plates. The titre is the dilution at which there is no longer sufficient antibody present to prevent or inhibit haemagglutination (Janke, 2000). When paired serology is used, ideally samples should be collected 2–3 weeks apart, and if there is at least a 4-fold increase in titre levels between the two samples, this is interpreted as SIV infection (Janke, 2000). In vaccinated herds the interpretation of the serological results in the breeding herd is almost impossible due to the cross reactions that can occur between the HA-antigens.
Treatment
As SIV is a viral disease, there is no treatment available and the pigs will usually recover within a week without treatment. Paracetamol or aspirin may be used to assist with recovery and act as support therapy (White, 2006). As previously mentioned, SIV can increase the susceptibility of pigs to other diseases such as A. pleuropneumoniae particularly seen in grower pigs. These secondary infections can be treated with antibiotics in order to reduce the substantial losses which can occur from such diseases (White, 2006).
Prevention
Biosecurity and hygiene
An important way to prevent and minimise the transition of SIV is by having excellent biosecurity practices in place (Torremorell et al, 2012). Disinfection of buildings and equipment should be carried out to ensure that the virus is not spread through the farm or to new batches of animals moving into accommodation. Herds that are sero-negative should only introduce animals with the same health status to prevent outbreaks in the unit. To completely remove the virus from enzootically infected herds, depopulation and restocking must occur (Taylor, 1995).
Attention should also be paid to the introduction of gilts into a herd, as replacement gilts positive to other SIV strains is a wellrecognised potential route of virus transmission.
Replacement animals should be kept in quarantine and remain isolated from the rest of the herd until they have been tested to prevent pigs which are possibly carrying influenza coming into contact with herds that have not been exposed to the virus yet (Jilani et al, 2019). Bird proofing buildings is also a good way of preventing avian species and their excretion from getting into contact with pigs but this is oft en difficult to achieve, and impossible to achieve for outdoor producers (White, 2006).
Minimising exposure of pigs from different age groups as well as other pigs that may be brought into the unit is important. It is also important to minimise exposure of people to pigs, particularly if they have flu-like symptoms. Prevention plans could include the vaccination of farm workers with seasonal flu vaccines, the restriction of visitors and the implementation of a shower in/shower out policy (Torremorell et al, 2012).
Vaccination — generating immunity
Vaccination is an intervention that can be used to control the impact of SIV on farm. It is important to remember that vaccination does not prevent infection, but it can reduce the clinical signs, lung lesions and the viral shedding (Lee et al, 2007). Vaccination initiates an adaptive immune response to SIV, which includes both humoral and cell-mediated immunity. Humoral immunity involves the production of antibodies against a particular pathogen and can be induced by both live and killed organisms which enter the body. Cell-mediated immunity involves cytotoxic T cells which can recognise and destroy infected host cells, therefore preventing the further spread within the body. Lymphocytes are involved in both mechanisms and are able to recognise antigens on a virus resulting in a response, and some also form memory cells which results in a quicker and stronger response if infection occurs for a second time (Sandbulte et al, 2015).
Vaccination — which vaccine and which animals?
Vaccine efficacy is affected by the homology between the vaccine and the challenge strain, the immunogenicity of the vaccine components and the antigen and adjuvant used in the vaccine (Sandbulte et al, 2015). Selecting the correct vaccine is extremely important as many new strains have formed over the last 20 years due to various types of mutation to the proteins on the virus' membrane. It is particularly important to ensure that the vaccine matches the HA protein on the challenge strain, as this is the protein that the vaccine is required to generate antibodies against (Grebe et al, 2008). There are a few commercial vaccines available on the market, for both the most current subtypes of SIV; H1N1, H1N2, H3N2 and pandemic strains.
In order for successful vaccination implementation, each farm needs to know the virus status and immune profile at the pig level and within different age groups (Vincent et al, 2017). The most common vaccination protocol is by vaccinating sows prior to farrowing, aiming to reduce infection pressure on the unit and therefore clinical cases. However, if influenza is a problem in the growers or finishers, piglet vaccination may be more beneficial (Torremorell et al, 2012). A complete economic analysis should be carried out that considers performance, morbidity and mortality, which will determine the financial benefit of vaccinating the growing herd.
Vaccination — sows
The likelihood of infection with influenza virus is increased during pregnancy due to alterations of the maternal immune system (Littauer et al, 2017). A reduction in progesterone synthesis in the corpus luteum in pregnant animals may occur as a result of the infection (Kim et al, 2014). This low progesterone level can consequently induce luteolysis and therefore termination of the pregnancy (Diehl and Day, 1974). A field study completed by Gumbert et al (2019) evaluated the reproductive performance parameters in sow herds infected with pandemic influenza A virus, following the implementation of Respiporc FLUpan H1N1 (Ceva Animal Health Ltd). Clinical signs were recorded in 129 farms prior to vaccination and reduced reproductive performance was observed in 79.8% of farms. A significant reduction (p<0.001) in return to oestrus was seen in 74.8% of farms following the implementation of vaccination. The mean return to oestrus rate in these farms reduced by 5.1%. The higher the return to oestrus was prior to vaccination, the greater the difference seen following vaccination. The abortion rate decreased by 1.8% (p<0.001) on average in 57% of farms following vaccination and the number of piglets born alive increased by an average of 0.6 piglets (p=0.001) in 70% of farms included in the study.
Sow vaccination is also carried out with the aim of transferring maternal IgA, IgG and IgM antibodies to SIV to the piglets (Cador et al, 2016a). Piglets with maternally derived antibodies (MDAs) have reduced susceptibility to influenza infection, however MDA presence has also been shown to increase the duration of outbreaks due to increased transmission between batches, therefore increasing the chances of prolonged influenza problems on the unit (Cador et al, 2016b). Another study investigated the impact of MDA on responses developed in pigs following a primary H1avN1 infection and the consequent protection acquired against a second homologous infection occurring 4 weeks later. For this, responses measured in MDA+ animals first inoculated at different ages (5, 7 and 11 weeks) were compared with those occurring in MDA-animals. The study found that MDA can offer clinical protection against swine influenza, as 5-week old high MDA+ pigs were clinically protected vs 7-week and 11-week old pigs which showed signs of hyperthermia. However, it was also concluded that passive immunity, regardless of MDA level, did not prevent the replication and shedding of the virus (Deblanc et al, 2018). Therefore, although vaccinating sows generates some piglet protection, it may still be required to vaccinate piglets to stop virus transmission on a unit. When vaccinating piglets, it is important to remember that MDAs can interfere with the development of active immunity following vaccination (Salmon et al, 2009).
Conclusion
Swine influenza is an important disease in the pig industry and can result in large economic losses due to performance reduction in both the breeding stock and the growing pigs. Biosecurity and hygiene measures should be part of the farm control plan for SIV and can be effective in preventing the introduction of virus as well as the spread within the farm if already present. Vaccination with products containing inactivated virus can be successful at generating high titres of serum antibodies which provide protection against SIV. It is important to ensure the right vaccine is used dependent on the strains causing the disease on the farm, as determined by diagnostic testing.
KEY POINTS
- Swine influenza A virus (SIV) can have a large economic impact on the pig industry.
- New subtypes can develop by antigenic shift, antigenic drift or reassortment.
- Diagnostic method should be selected based on the clinical picture and the age of the affected animals.
- Biosecurity and hygiene are very important in preventing and minimising the transition of influenza, including disinfection of buildings and equipment and quarantining of new gilts.
- Vaccination can be very effective in controlling the impact of SIV on farm and it is important to ensure the correct vaccination protocol is in place, based on the individual farm case.