The clinical importance of diarrhoea in calves means that the gastrointestinal mucosa is of particular interest to veterinarians. This article looks at the structure and function of the gastrointestinal mucosa and the development of the gastrointestinal microbiota, followed by strategies for optimising gastrointestinal mucosal immunity, including the use of colostrum, enhancing enteropathogen-specific antibodies and supporting the microbiota.
The gastrointestinal mucosa
The mucosa is the largest component of the immune system, providing a first line of defence against encountered pathogens. The gastrointestinal mucosa is highly specialised and, uniquely, needs to balance immune and digestive functions. It comprises three primary layers: a mucus barrier, an epithelial cell layer and the lamina propria (Figure 1). Together, these layers form an effective defence against enteropathogens termed the ‘mucosal firewall’ (Chase and Kaushik, 2019).
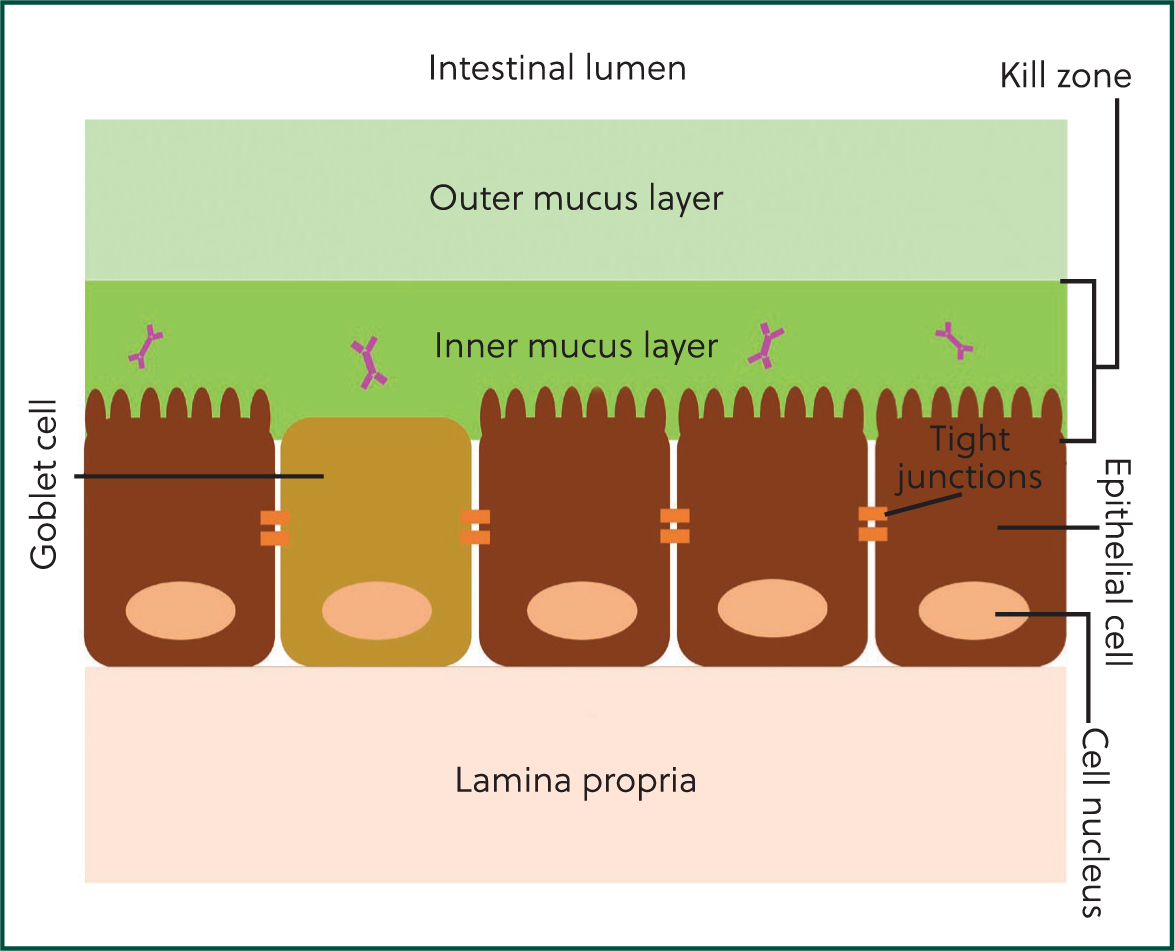
The mucus barrier
The inner layer of the mucus barrier is made up of a dense proteoglycan gel created by the combination of mucin and mucus produced by goblet cells (specialised epithelial cells). Antimicrobial peptides and antibodies, such as secretory IgA, are concentrated here, generating effective protection against pathogen incursion. Collectively this layer (comprising mucus and antimicrobial compounds) is termed the ‘kill zone’ (Chase and Kaushik, 2019).
The outer layer of the mucus barrier is less dense in structure than the inner layer and is colonised by the microbiota. Together, the inner and outer mucus layers protect the underlying epithelial cell layer and provide an effective physical barrier against pathogens, contributing to the innate immune system. Innate immunity is the body's initial defence mechanism against pathogens and primarily comprises of defensive barriers, including the mucosa. Innate immunity provides a rapid response but is not pathogen specific and does not retain any immunological memory for pathogens. By contrast, while slower than innate responses, acquired (or adaptive) immunity provides a pathogen-specific response through antibody-producing cells and retains immunological memory in the event of repeat infections with the same pathogen. The mucus barrier interacts with both immune systems by providing a physical barrier (innate immunity) and hosting antibodies (acquired immunity) in the kill zone, an example of the complementary nature of the innate and acquired immune systems.
The epithelial cell layer
The epithelial cell layer is a monolayer of cells that play a crucial role in both digestion and the organisation of local enteric immune responses to ingested pathogens (Maynard et al, 2012). Several types of epithelial cells are present in this layer, each with a distinct function (Table 1).
Table 1. Main cell types present in the gastrointestinal epithelial cell layer
Cell type | Function |
---|---|
Enterocytes | Digestive cells primarily involved in absorption of nutrients |
Enteroendocrine cells | Secrete gastrointestinal hormones involved in digestion, appetite and insulin regulation, such as cholecystokinin and glucagon-like peptide 1 (Gribble and Reimann, 2019) |
Goblet cells | Secrete mucus and mucin to form the mucus layer |
M cells (also called microfold cells) | Cells that are primarily involved in antigen uptake |
Paneth cells | Secrete antimicrobial peptides into the inner mucus layer |
The cells in the epithelial layer continuously interact with the microbiota in a reciprocal fashion (Figure 2); for example, while one function of the epithelial cells is to prevent excessive over-growth of microbiota populations, organisms within the micro-biota secrete products that promote the release of mucus from goblet cells, thus maintaining the mucus layer that protects the epithelial cells (Maynard et al, 2012).
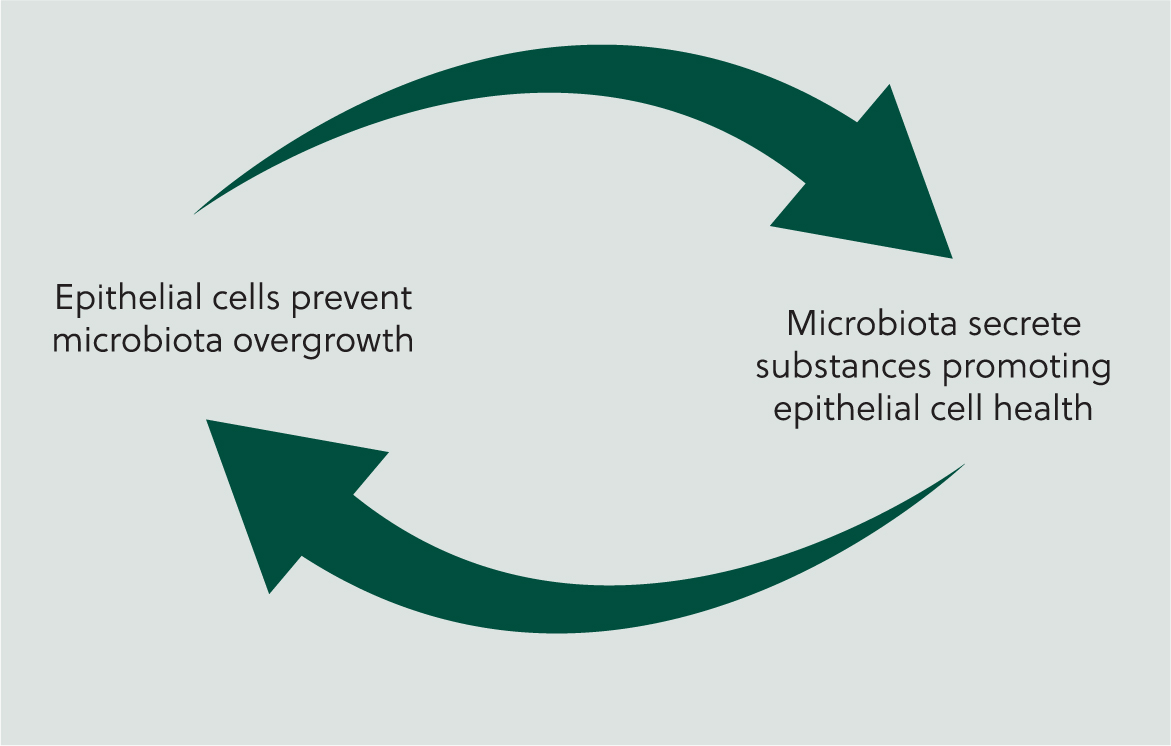
The lamina propria
The lamina propria is a thin layer of connective tissue that supports the epithelial cell layer, allowing flexibility and movement of the epithelium, as well as contributing to innate and acquired immune responses. Secretory immunoglobulin A (sIgA) in the gastrointestinal tract is produced by plasma cells that reside in the lamina propria before migrating from the lamina propria to the inner mucus layer. Here, sIgA is involved in gastrointestinal immune defences and contributes to maintenance of the commensal microbiota (Macpherson et al, 2001). Additional immune responses to pathogens that breach the mucus barrier and epithelial cell layer and reach the lamina propria are provided by phagocytic cells and immune proteins such as cytokines and complement proteins (Maldonado-Contreras and McCormick, 2011). Additionally, immune cells that form part of the acquired immune system (such as T-cells) can also be recruited to the lamina propria, providing a pathogen-specific defence in cases of repeat infection (Maldonado-Contreras and McCormick, 2011; Chase and Kaushik, 2019).
Gastrointestinal microbiota
Mucosal health depends on the presence of a healthy and diverse community of microorganisms (termed the microbiota (Box 1)), including bacteria, yeasts and viruses (Rinninella et al, 2019). Furthermore, maturation of the mucosal immune system depends on exposure to microorganisms (Cortese, 2009). The microbiota of newborn calves undergoes rapid change in the first few days of life (Klein-Jöbstl et al, 2019): facultative anaerobes initially dominate but, as oxygen present in the fetal gastrointestinal tract becomes depleted in the first few days of life, the microbiota transitions to a community where obligate anaerobes predominate (Gomez et al, 2019; Klein-Jöbstl et al, 2019). After approximately 4 weeks the calf microbiota begins to stabilise and differences in the relative abundance of microorganisms are observed depending on the location sampled (Dias et al, 2018). For example, Prevotella spp. predominate in the rumen microbiota, with Lactobacillus spp. occurring at low prevalence in this region, whereas the opposite is observed in jejunal samples (Dias et al, 2018; Robles-Rodríguez et al, 2023).
Box 1.Definitions relating to commensal microorganism communities
- Microbiota: the community of microorganisms in a defined environment (eg, the gastrointestinal tract)
- Microbiome: the collection of genomic material from all the microorganisms in a defined environment
- Richness: number of microorganism species present in the microbiota
- Evenness: the relative differences in the abundance of different microorganism species in the microbiota
- Diversity: the number of distinct species of microorganisms and their abundance in the microbiota (diversity is a combination of richness and evenness)
Colonisation and establishment of the gastrointestinal microbiota
Historically, it has been believed that the healthy in utero environment is sterile, with microbial colonisation of the neonate first occurring at birth (Alipour et al, 2018), but findings of studies performed in the past decade have challenged this hypothesis. Results of recent studies suggest that colonisation of the neonatal microbiota may start when the fetus is still in utero (Collado et al, 2016; Alipour et al, 2018; Barden et al, 2020; Zhu et al, 2021); a hypothesis supported by studies finding that bacteria are present in the gastrointestinal tract when calves are less than 30 minutes old (Malmuthuge et al, 2015; Klein-Jöbstl et al, 2019), sooner than would be expected if colonisation started during birth. However, it should be considered that studies are often limited by difficulties encountered when measuring the in utero microbial environment (avoiding external contamination can be particularly challenging) and further research is needed (Alipour et al, 2018; Husso et al, 2021).
Development of the gastrointestinal microbiota
While the source of initial colonisation is disputed, it is widely agreed that post-birth development of the enteric microbiota is influenced by both internal and external factors, such as calf nutritional state, gastrointestinal functions and exposure to environmental micro-organisms (Figure 3). During and shortly after birth, calves are exposed to their dam's vaginal, faecal and oral microbiota. Studies have found that the newborn calf gastrointestinal microbiota is similar to their dam's oral and vaginal microbiota, but interestingly the dam faecal microbiota has been found be less influential (Alipour et al, 2018; Klein-Jöbstl et al, 2019; Barden et al, 2020). In humans it is well established that the gastrointestinal microbiota of newborn babies born via caesarean section differs to babies born per vaginum (Dzidic et al, 2018; Zhang et al, 2021) but the effect of birth experience on the microbiota of neonatal calves is poorly studied. A single study found that small numbers of bacteria were present in the amniotic fluid and meconium of calves born via caesarean section, but the authors concluded that the bacterial quantities present were clinically insignificant and unlikely to contribute to the gastrointestinal microbiota (Husso et al, 2021). These findings suggest that the gastrointestinal microbiota of calves born via caesarean section may differ from other calves; however, Husso et al (2021) did not include calves born per vaginum in their study for comparison and more work is needed to explore this further.
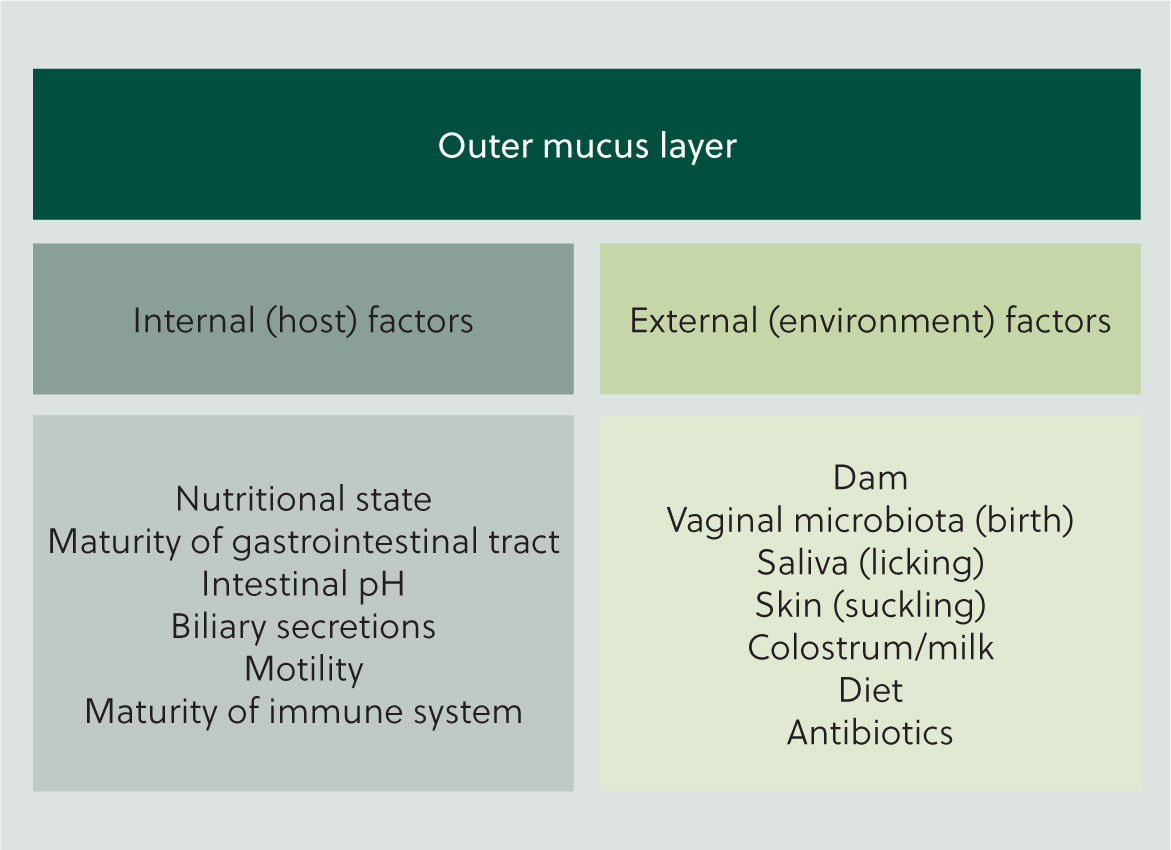
Stressful events such as disbudding and castration have been found to negatively affect the gastrointestinal microbiota of older calves (Mir et al, 2019) and it is hypothesised that stress likely has similar effects on the microbiota of neonatal calves (Osorio, 2020). While this is a plausible hypothesis, the paucity of existing data means the effects of stress on the bovine neonatal microbiota is currently unclear. Nevertheless, it is known that both maternal and fetal stress have an impact on neonatal gastrointestinal development in a wider sense (Osorio, 2020) and it is prudent to minimise stress as far as possible.
Colostrum is also thought have a significant role in the development of the gastrointestinal microbiota in neonatal calves, but existing data are conflicting (Yeoman et al, 2018; Klein-Jöbstl et al, 2019). The relationship between colostrum and gastrointestinal microbiota is discussed further later in the article.
Optimising gastrointestinal mucosal immunity
Globally, neonatal calf diarrhoea is the leading cause of mortality and morbidity of calves under 1 month of age (Meganck et al, 2014) and optimisation of neonatal immune defences (together with minimisation of enteropathogen challenge) plays a key preventative role. As immune responses against enteropathogens occur at the mucosal level, strategies should focus on measures that will support the gastrointestinal mucosa and enhance mucosal immune defences.
Colostrum
It is well known that optimisation of colostrum management on farms is crucial for immunity of neonatal calves. Detailed description of colostrum management strategies is beyond the scope of this article but is well described elsewhere (eg Godden et al, 2019; Geiger, 2020).
Influence of colostrum on calf gastrointestinal microbiota
Colostrum is thought to be important for the developing gastrointestinal microbiota in young calves as it is both a source of microorganisms that may contribute to the microbiota and of carbohydrates that promote microorganism growth. However, Klein-Jöbstl et al (2019) found that the faecal microbiota of calves in the first 48 hours of life was not influenced by colostral micro-organisms, suggesting that colostrum may not be a primary contributor to the neonatal gastrointestinal microbiota. By contrast, Yeoman et al (2018) identified similarities between calf gastrointestinal and colostral microbiota that could be explained by the consumption of colostrum. The role of colostrum in development of the bovine neonatal gastrointestinal microbiota is not yet fully understood and further work is needed; nevertheless, the immunological importance of colostrum is well established and accordingly, it is essential that colostrum management is optimised to ensure adequate immunoglobulin transfer.
Although results of studies investigating the role of colostrum in development of the calf gastrointestinal microbiota are conflicting, colostrum management has consistently been found to affect the neonatal microbiota. Colostrum-deprived calves have been found to have fewer intestinal bacteria overall compared to calves that consumed colostrum shortly after birth (Malmuthuge et al, 2015) and delayed colostrum feeding (12 hours after birth compared to 45 minutes) has been associated with a lower intestinal prevalence of Bifidobacterium spp. and Lactobacillus spp. (Fischer et al, 2018a). The microbiota of colostrum-deprived calves has also been found to have a higher proportion of Escherichia coli and a lower proportion of Bifidobacterium spp. compared to calves that received colostrum (Malmuthuge et al, 2015). While heat treatment of colostrum is typically performed to reduce the numbers of pathogenic bacteria present (eg Godden et al (2012)), heat treatment may also be beneficial for the gastrointestinal microbiota. Studies are few, but increased prevalence of Bifidobacterium spp. and increased availability of oligosaccharides to support microbiota growth have been associated with feeding of heat-treated colostrum (Malmuthuge et al, 2015; Fischer et al, 2018b). Further work is needed, but present data suggest that heat treatment of colostrum may have additional benefits beyond the reduction of potentially harmful bacteria.
Relationship between colostrum and gastrointestinal mucosal antibodies
Antibodies are immunoglobulins that are unbound from plasma cells (antibody producing cells); accordingly, immunoglobulins are necessary for antibody-mediated immune responses to be able to occur (Marshall et al, 2018). The synepitheliochorial structure of the ruminant placenta prevents transplacental transfer of immunoglobulins to the fetus, therefore colostrum is an essential source of immunoglobulins for newborn ruminants. Immunoglobulin G (IgG) is the predominant immunoglobulin in bovine colostrum, comprising 85–95% of the immunoglobulin component (Table 2): two subtypes of IgG (IgG1 and IgG2) are present, with IgG1 making up 80–90% of the IgG component of bovine colostrum (Ahmann et al, 2021).
Table 2. Immunoglobulins in bovine colostrum
Immunoglobulin type | Proportion of total immunoglobulin fraction of colostrum | Function |
---|---|---|
IgA | ~5% | Primarily involved in intestinal immunity |
IgG | 85–95% | Early life immunity via passive transfer of immunity (PTI) |
IgM | ~7% | Contributes to defences against septicaemia and bacteraemia |
Circulating IgG1 is re-secreted from the bloodstream into the calf 's intestinal lumen at a rate of 2–4g per day for the first 2 weeks of life (Besser et al, 1987; Besser et al, 1988a); IgG2 is not re-secreted, a finding that may explain the predominance of IgG1 in bovine colostrum. It is thought that the re-secretion of IgG provides support to the gastrointestinal mucosal immune defences in the first two weeks of life as, while sIgA is the primary immunoglobulin involved in gastrointestinal mucosal immunity (Maldonado-Contreras and McCormick, 2011), production of sIgA by neonates is low (Besser et al, 1988b; Ulfman et al, 2018). This hypothesis is supported by results of a study that found that IgG1 was the primary rotavirus antibody present in the gastrointestinal lumen of 5- and 10-day-old calves fed colostrum harvested from vaccinated cows (Besser et al, 1988b), suggesting that re-secreted IgG1 is capable of providing enteropathogen-specific immunity. Furthermore, the same study found that serum and intestinal IgG concentrations were closely correlated (Besser et al, 1988b); therefore, measurement of serum total protein (typically used as an indicator of serum IgG) is likely to be a reliable proxy indicator of intestinal IgG concentration.
Enhancing enteropathogen-specific antibodies
Vaccination
Vaccination can be a valuable tool for enhancing enteropathogen-specific antibody responses and is frequently employed in neonatal calf diarrhoea prevention strategies (Maier et al, 2022). Commonly, cows are vaccinated in late pregnancy with the aim of enhancing colostral antibodies to neonatal calf diarrhoea pathogens (Crouch et al, 2000) (eg rotavirus or coronavirus vaccines), although administering vaccinations at the correct time can be challenging on farms where accurate calving dates are not known, which may limit vaccine efficacy. Vaccines administered directly to the calf are also available but these are typically used for pathogens causing diarrhoea in older calves (eg salmonella vaccines). Vaccines that enhance pathogen-specific colostral antibodies can support gastrointestinal mucosal immunity by increasing the fraction of re-secreted IgG1 that is specific to enteropathogens likely to be encountered by the gastrointestinal mucosa (eg rotavirus). These types of vaccines are preferred in prevention strategies aimed at neonatal calf diarrhoea pathogens because affected calves are very young (less than 1 month old) and maternally derived antibodies do not interfere with this method of vaccination (Maier et al, 2022), rather, this method of vaccination depends on the presence of enteropathogen-specific maternally derived antibodies in colostrum. Therefore, this type of vaccination depends on the dam mounting an effective antibody response following vaccination for there to be a corresponding effect on colostral antibody concentration. While generally considered a reliable response, variations are reported, with some studies finding that different vaccines induce different antibody responses (eg Gonzalez et al, 2021). It also needs to be considered that this type of vaccination inevitably depends on good colostrum management on farm to be effective. Accordingly, if failure of transfer of passive immunity (FTPI) prevalence is high on a particular farm, these types of vaccines are unlikely to be a useful aid in the prevention of neonatal calf diarrhoea until colostrum management is improved.
Oral passive immune therapy
Oral administration of enteropathogen specific IgG has been suggested as a possible preventative strategy for neonatal calf diarrhoea that may offer the benefits of dam vaccination (provision of maternally derived enteropathogen-specific antibodies), while remaining independent of colostrum management (the product is administered directly to the calf). The mechanism of action of these products is currently unclear; however, it has been hypothesised that oral administration of enteropathogen-specific IgG supplements IgG gained through colostrum consumption and enhances the enteropathogen-specific IgG1 available for resecretion (Nussbaum et al, 2023). However, while these types of product have been commercially available for several years, independent studies are few and results are conflicting. Nussbaum et al (2023) recently reported that the odds of developing diarrhoea was reduced in calves treated with a product containing E. coli, coronavirus, and rotavirus-specific IgG in addition to the farm's standard colostrum protocols when compared to calves that received colostrum alone. Similarly, Vega et al (2011) reported diarrhoea onset was delayed and diarrhoea severity and duration was reduced in calves treated with a product containing antibodies specific to rotavirus, coronavirus, E. coli and Salmonella spp. By contrast, Chung et al (2019) did not identify any beneficial effect of passive immune therapy, a finding that may be explained by differences in study design, as calves were only recruited if they were observed to have diarrhoea, whereas Nussbaum et al (2023) and Vega et al (2011) treated calves within 24 hours of birth, before diarrhoea developed.
Supporting the microbiota
There is increasing interest in the use of probiotic and prebiotic products in the prevention of calf diarrhoea. These products are typically added to food and aim to either supplement the calf 's gastrointestinal microbiota with live strains of microorganisms beneficial to health (probiotics) or provide a substrate to promote the growth of favourable host microorganisms (prebiotics) (Cangiano et al, 2020).
Common probiotics available for treatment of calves include yeast-based and bacteria-based products. Yeast-based products can be live yeast (dried living yeast) or yeast cultures (products of yeast fermentation); yeast cultures act as both a pro- and a pre-biotic (although are classified as probiotics). Bacteria-based probiotics use bacteria known to be beneficial to health such as Lactobacillus spp. and Bifidobacterium spp. and may limit pathogen invasion by promoting gastrointestinal microbiota health and enhancing mucosal immunity (Cangiano et al, 2020; Villot et al, 2020). Oligosaccharides (simple sugars occurring in substances such as milk and colostrum) are the primary prebiotics studied for treatment of calves. Oligosaccharide prebiotics have been found to have a beneficial effect on the health of newborn babies (Srinivasjois et al, 2013) but results are more mixed in neonatal calves.
There are many studies investigating the effects of pro- and pre-biotics on calf health and productivity and these have been extensively reviewed elsewhere (eg Alawneh et al, 2020; Cangiano et al, 2020; Du et al, 2023). Generally results are conflicting, with studies frequently reporting no effect; however, negative effects are not reported, and some beneficial effects are reported across studies, primarily in the areas of gastrointestinal health and calf growth. Improvements in measures of gastrointestinal mucosal immune function are also reported (Villot et al, 2020). Beneficial effects of pro- and pre-biotic treatment on calf growth and gut health have also been identified in meta-analyses (Alawneh et al, 2020; Wang et al, 2023), although a high risk of study bias has been reported (Alawneh et al, 2020) and continued work is warranted to enable more definitive conclusions to be drawn.
Conclusions
The mucosa is the largest organ of the immune system. Collectively, the three layers of the mucosa form an almost impenetrable barrier – providing a highly effective first line immune defence against pathogens. Optimisation of gastrointestinal immunity is a key aspect of prevention strategies for calf diarrhoea and in practice can be achieved through educating farmers on effective colostrum management, maximising enteropathogen-specific antibody immunity through appropriate vaccination and oral passive immune therapy, and supporting the gastrointestinal microbiome.
KEY POINTS
- The mucosa is the largest component of the immune system
- The gastrointestinal mucosa is highly specialised with digestive and immune functions
- The mucosa comprises three layers that collectively form a ‘mucosal firewall’, providing a highly effective immune defence against pathogens
- Optimising gastrointestinal mucosal immunity is a key aspect of calf diarrhoea prevention strategies