The National Food Strategy calls on the countryside to be utilised to ‘sequester carbon and restore nature’ (Dimbleby et al, 2021), by encouraging diverse methods of land management. With grasslands or pasture currently occupying 70% of the globe's total agricultural area (Ramankutty et al, 2008; Bengtsson et al, 2019), how this resource is managed in the future will have large impacts on farming's environmental impact. Grassland co-evolved with herbivores over millions of years to create carbon-rich soils (Follett and Reed, 2010), however current estimates suggest over half of their soil carbon has been lost (Sanderman et al, 2017). Consequently, their once net cooling effect on the globe has been superseded by a net warming effect (Chang et al, 2021). This transition from a greenhouse gas sink to source is attributed to land conversion from natural ecosystems to grasslands, grasslands to arable use, and an intensification of pasture for livestock production (Follett and Reed, 2010). The International Plan for Climate Change recognises the need for improved carbon sequestration associated with food production, estimating that 89% of all agricultural greenhouse gas production could be mitigated through changes in farm practices (Smith et al, 2008).
In tandem, at the time of writing and for the foreseeable future, we are experiencing unprecedented market volatility for the 3 ‘f 's’ of farming: food, fuel and fertiliser. Economic necessity is driving farmers to question all their inputs and this, in combination with a wider societal and political realisation regarding agriculture's true environmental impact and individual farmers' own will to become more economically resilient and farm in a manner they deem to be more ‘sustainable’ in the future, has led many to become more receptive to alternative farming methods.
Regenerative agriculture offers one such approach that aims to farm more in harmony with nature, both above and below ground, and simultaneously provide food production and environmental stewardship. Farming with ecological integrity (Massy, 2020) aims to have a positive effect on biodiversity, maximising an agroecosystem's carbon sequestration and producing both quality nutrition and an alternative route to farm profitability. The minimal external inputs aids economic resilience (White, 2020), while the requirement for a regenerative system to buy in minimal or no livestock feed has far-reaching environmental benefits (Al-Kaisi and Lal, 2020).
UK agriculture does not want the production of the inputs for its farming to be detrimental elsewhere in the world, known as ‘ghost acres’ (the land abroad that is used to grow feed for animals within a country). At present 80% of the UK's imported soya is destined for animal feeds (Coleman et al, 2021).
While profitability has potential to be improved as a result of a lowered cost of production model associated with a regenerative approach, further data still need to be collated regarding total outputs in comparison to other farming systems. Within grain production, regenerative acreage produced 29% less in comparison to ‘conventional’ grain production (LaCanne and Lundgren, 2018). Within organic animal husbandry agriculture lower outputs are recognised (Durham and Mizik, 2021), and it would be expected for regenerative agriculture to be comparable to this. The strengths and weaknesses of the multiple competing farm systems is recognised within the National Food Strategy by the prediction of ‘lower intensity, agroecological’ farm enterprises to sit alongside ‘higher-yielding farms that use the latest technology to maintain yields without polluting’ (Dimbleby et al, 2021).
Regenerative agriculture
There is no singular, approved definition of regenerative farming, as highlighted by a review of 28 different studies (Schreefel et al, 2020). Robert Rodale (1930–1990) first coined the term ‘regenerative organic’ to distinguish a holistic farming approach that goes beyond simply sustainable (LaSalle et al, 2008). It acknowledges the interconnected reality of any healthy ecosystem, with no one action within nature's complex adaptive systems occurring in isolation.
Regenerative agriculture's outcome-based approach is underpinned by six practices. At its crux is the understanding that the health of the entire food system is intrinsically linked to soil health. These practices are — the limited disturbance of soil, whether physically, chemically (synthetic fertilisers) or mechanically (tillage); the requirement for constant vegetation covering soils, with living roots within the soil for as long as possible each year, and the absolute avoidance of bare soils. A biodiversity of plants and animals is encouraged. In contrast to post World War II farm specialisation (Robinson and Sutherland, 2002), livestock grazing is integrated within the system. The sixth practice is context — referring to the fact that no two farms or fields are identical, ie. the approach for two different farms will likely differ (Figure 1).
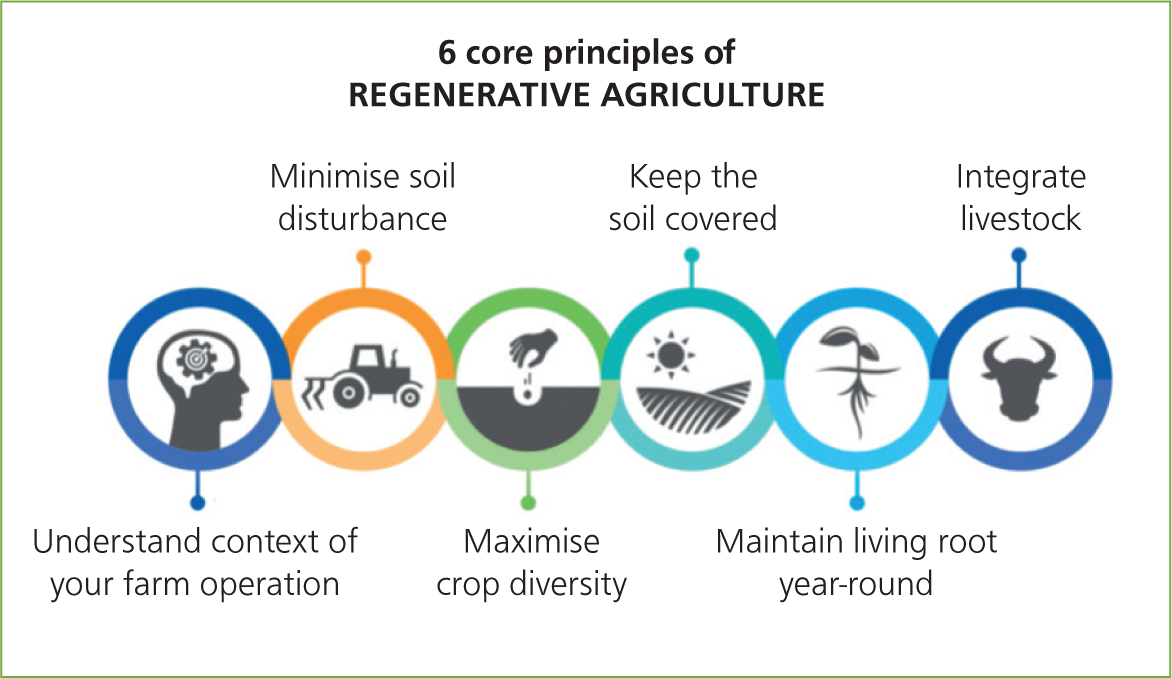
In short the practices are:
- Minimise soil disturbance
- Maximise crop diversity and encourage farm biodiversity
- Maintain soil cover
- Maintain living roots throughout the year
- Integrate livestock
- And understand farm context (Regenerative Agriculture, 2020).
A biologically active soil is regarded as the engine of the farm ecosystem, and with active management the soil will improve in both its quality and the ability to sequester carbon (Brown, 2018). It will also support a greater biodiversity both above and below ground. Soil's importance is emphasised by 90% of living organisms within terrestrial ecosystems spending at least a portion of their life cycles in soil habitats (WWF, 2020). Although the nutrients may be within the soil, without functional bacteria or mycorrhizal fungi, plant life does not have the ability to efficiently access these nutrients. At present soil is being destroyed at 10 times faster than it is created (Maximillian et al, 2019). Specific to the UK, although our islands only occupy 1% of Europe's land mass, we account for 5% of the continent's soil erosion by water (Panagos et al, 2015). And specific to South West England is the visible increased surface run-off associated with the degraded soils of farmland (Palmer and Smith, 2013). Also pertinent to the South West is the tripling of the area used to grow maize since the early 1990s (Farnworth and Melchett, 2015).
Fields where maize is grown are subject to high rates of erosion because of shallow roots failing to bind the soil, the lack of under sowing that occurs with maize cultivation, and by the timing of harvesting (late autumn). Where maize is grown, up to half of river sediment can come directly from maize fields (Figure 2) (Mokhtar, 2010).
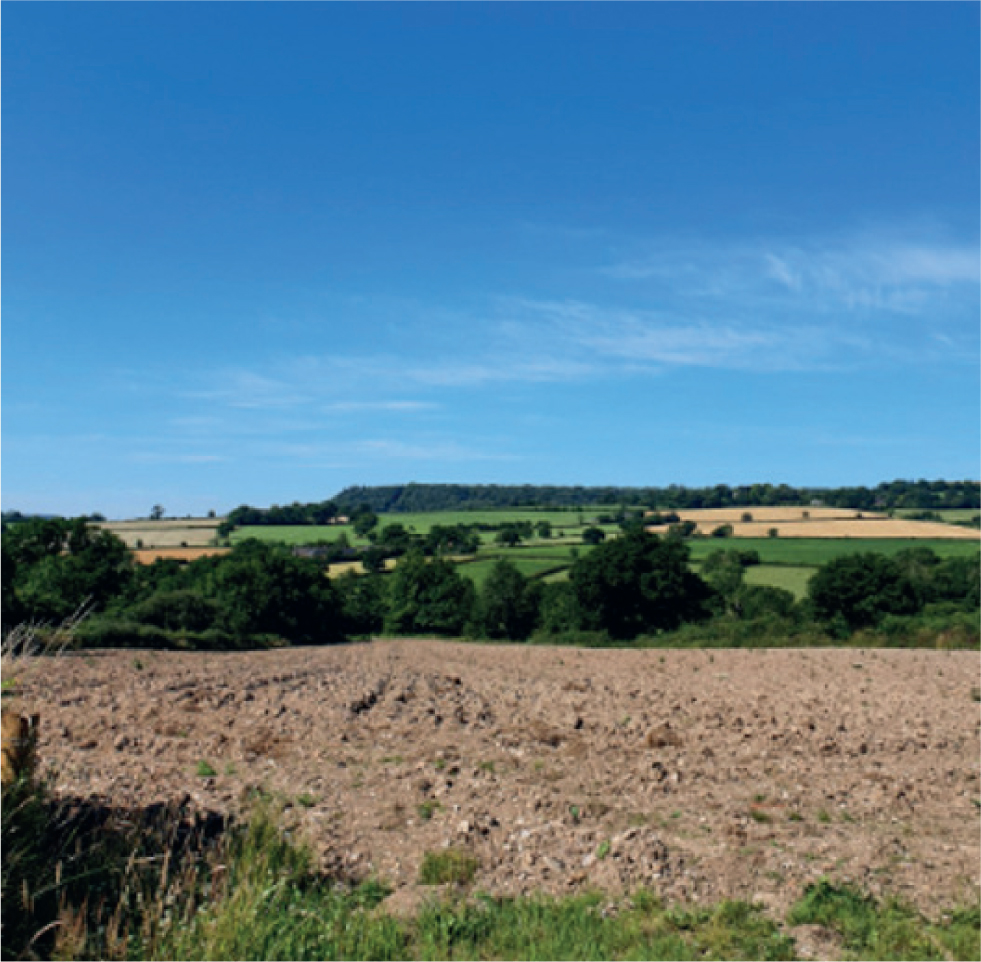
One spoonful of healthy soil should contain more bacteria than there are people on Earth, but also (invisible to the naked eye) mycorrhizal fungal filaments that can total up to several miles in length. An active soil should also contain prey and predator species of nematodes, arthropods and what Aristotle described as ‘the intestines of the soil’, the earthworm. On a larger scale, 1 m3 of soil should contain 25 000 kms of hyphae. Without them, only one thousandth of the surface area for mineral and nutrient absorption is provided for the plant root (Jansa et al, 2013).
Regenerative agriculture principles
Healthy soil is composed of 50% minerals, 25% water, 15% air and 10% soil organic matter. It is not uncommon for UK arable soils to contain as little as 1–2% soil organic matter (Muhammed et al, 2018).
Soil organic matter is the term used for all living, or onceliving, materials within, or added to, the soil. This includes roots developing during the growing season, incorporated crop stubble or added manures and slurries. All organic matter contains carbon (C), but it also contains nitrogen (N), phosphorus (P), sulphur (S), potassium (K), magnesium (Mg), calcium (Ca) and a whole range of micronutrients (e.g. copper (Cu) and zinc (Zn)). Soil organic matter can be estimated by multiplying soil carbon x 1.72 (Walkley Black method) (de Vos et al, 2007).
Walter Jehne describes healthy soils like cathedrals — the masonry is the mineral particles, the cement the soil organic matter, and the void within necessary for healthy soil to have sufficient surface area for both the uptake of essential minerals and nutrients and free-space for water storage. The glomalin produced by a functional mycorrhizal network is the glue that binds the soil aggregates (O'Brien, 2020). Individual roots should not be visible.
Regenerative agriculture aims to minimise soil disturbance either physically (over stocking, compaction), mechanically (the plough) or chemically (synthetic fertilisers). To advocate not ploughing, nor use of artificial fertilisers, is a dramatic shift from current agricultural norms. However, the answer regarding tilling is best considered by what nature would choose if left uninterrupted. The mechanical disturbance of removing the ground cover exposes a greater surface area of soil to air and sunlight (increasing wind and water erosion and also evaporation). The natural soil structure is compromised, reducing soil organic matter, and both soil biological and microbiological activity. 80% of a soil's bio-fertility relates to the surface exposure (Tibbett et al, 2020). Bare soils are particularly prone to compaction through precipitation, and for many parts of the globe, the radiating heat at ground level caused by a lack of cover will also promote a below ground soil temperature detrimental to functional soil microbes. Soil with minimal biology will further exacerbate compaction because of a lack of invertebrate aeration (Figure 3).
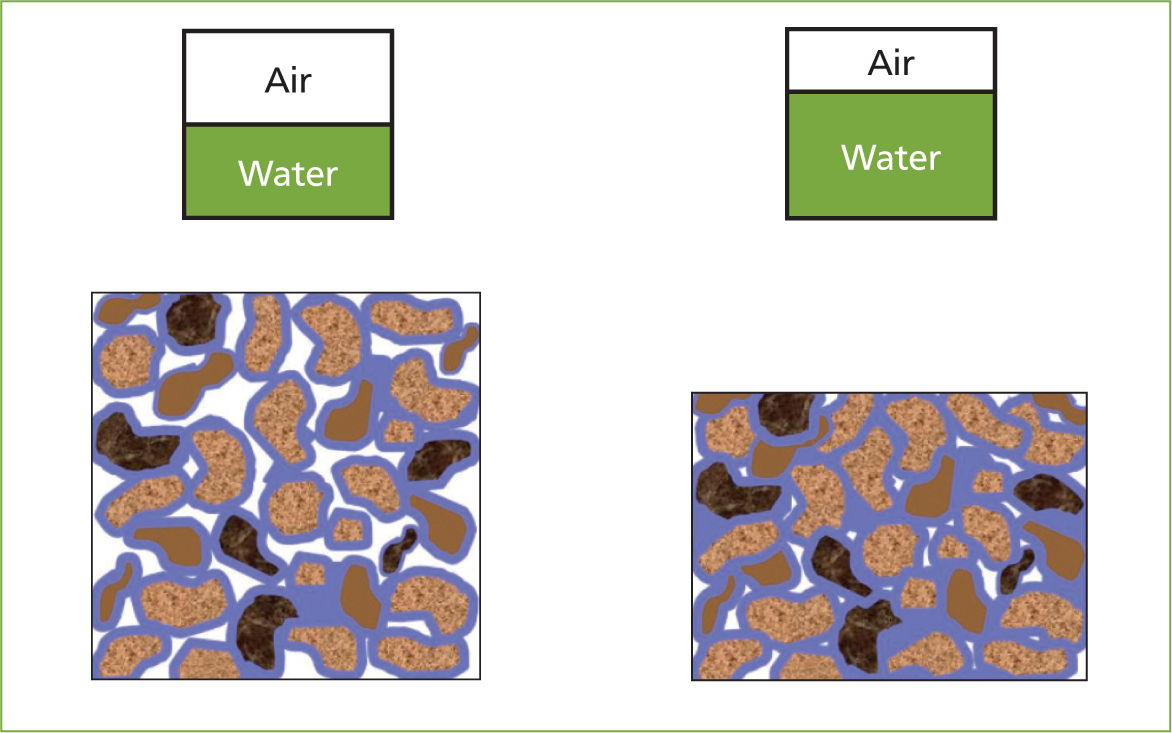
The pore spaces within the soil that provide the interface for nutrient transfer are compromised, with the disruption of physical networks (Young and Ritz, 2000). The subsequent change to soil microbial communities magnifies the degree of nutrient waste and run-off by altering key nutrient cycles (Smith et al, 2016). The frequent disturbances associated with tillage affects soil diversity by favouring species that tolerate disturbance better (Buckling et al, 2000). Evidence shows more diverse communities tend to be more stable in the face of environmental variation and more resistant to invasion by other species (Eisenhauer et al, 2012).
The water cycle is also interfered with by tillage, with water's ability to infiltrate soil being compromised. Soil that contains 1% more carbon, will store 140 000 litres more per hectare (Puthalpet, 2022). Rainfall penetration can be used as a key marker of soil health, with water penetrating the first inch in under 10 seconds and the second inch in under 20 seconds in the healthiest soils. Ideally, water will efficiently infiltrate soil profiles, and be stored within the soil until required by plants. No till soils are shown to have higher nutrient levels within the soils, and a greater number of DNA sequences associated with nitrogen fixation as a result of more active microbial communities. No till has been shown not to have a major impact on crop yield (DeFelice et al, 2006).
Synthetic fertiliser production, particularly nitrogen, is a potent producer of greenhouse gas emissions (Chai et al, 2019). Within the soil synthetic fertilisers suppress the role of nitrogen-fixing bacteria and enhance the role of everything that feeds on nitrogen. The decomposition of soil organic matter is amplified by changing the physical structure of soil. The destruction of the soil resource coupled with the lack of biodiversity seen within monocultures provides lower nutrient cycling. The solution to inadequate nutrient cycling has often been the addition of further synthetic fertiliser. The law of diminishing returns becomes the primary reason for a cessation in further additions. Synthetic fertilisers aid the propagation of weeds, as most flourish in a high nitrogen environment. With more weeds, there is the tendency to utilise more herbicides, many of which act as chelating agents, binding metals within the soil. Micronutrient availability becomes reduced to plants, increasing their disease susceptibility. While healthy plants may combat naturally occurring diseases, with increased disease susceptibility comes the increased use of fungicides. Fungicides in turn are detrimental to soil biology. With a lack of diversity and immunologically challenged plants, opportunistic pests can damage crops. Pesticides may then be used on crops, both those for direct human consumption, and those for livestock feed. Far from being selective, pesticides do not only work on the chosen insect, but also the predator insects which have evolved to take care of the pest species, as well as the pollinating insects critical to crop production. Entomologists give a conservative estimate of 1700 beneficial insect species for every recognised pest species (Lundgren and Fausti, 2015). It is a great illustration of the complex interconnecting web that nature has evolved, and by interrupting one pathway there are innumerate consequences.
While protecting the soil from erosion and evaporation, multi species cover crops, including triticale, rye, winter wheat, peas, sugarbeets, as well as a vast array of options and diverse pasture containing both grasses and broadleafs, optimise the collection of solar energy via photosynthesis. The resultant sugars are transferred into the plant roots, where they will feed the soil biome as root exudates. Cover crops can be selected based on their nutritional value to the soil, and while some may be allowed to be grazed by livestock, for other fields or farms it may be beneficial to allow them to decay within the soil, e.g. with nitrogen fixing legumes.
Regenerative agriculture aims to encourage the diversity of plant life, animal life and insects working together in synchrony to build a healthy ecosystem. Using a range of methods, be it planting and maintaining multi-species grass pasture, stacking enterprises with orchards with grazing ruminants below, letting sections of land grow unchecked, allowing flower-rich pollinators to grow, planting hedgerows and allowing them to flower, or fencing off woodlands and wetlands while incorporating wildlife belts within an integrated farm landscape, the aim is to reinvigorate natural ecosystems and make tangible impacts on ecosystem diversity.
Regenerative farming practices incorporate and integrate livestock within the system. The bovine is viewed as a valuable ecosystem tool, exchanging a mower for a mouth, an inoculator for a rumen (able to reintroduce microbes to the ground), a mobile fertilizing unit for a gastrointestinal tract and a compacter of organic material back into the ground as hooves (Matthews et al, 2018).
Adaptive multi-paddock (AMP) grazing aims to mimic how ancestral herds grazed the earth (Figure 4). Rather than set-stocking on pasture or grazing for long periods within a larger area (Byrnes et al, 2018), AMP uses both small paddocks to avoid selective grazing, and large numbers of cattle for short periods of time (hours to days). It can be described by the rule of thirds: eat a third, leave a third and trample a third into the ground, and is particularly efficacious in increasing soil organic matter (Teague et al, 2013). Grazing periods are followed by sufficient resting times (30–45 days in the growing season and up to 90 days in the non-growing season, compared with a standard 21 day rotation). At present McDonald's UK and one of the authors (AM) are piloting a project alongside FAI Farms to gain insights into what AMP grazing may look like within the commercial UK beef system, simultaneously developing a suite of metrics to assess progress towards healthy farming ecosystems.
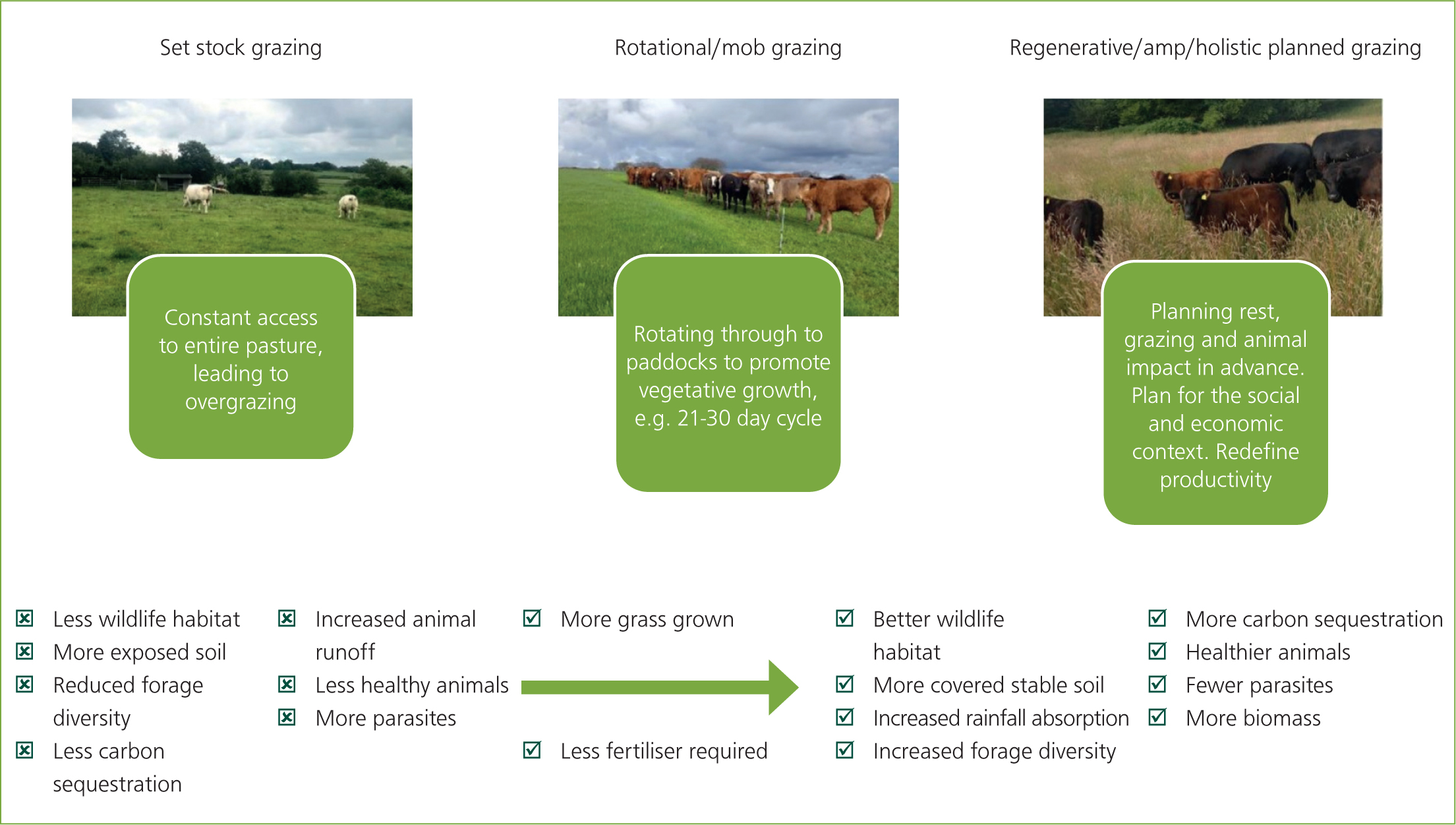
The final principle is context. What works in one location, because of its unique ecological circumstances, may not work in another location. Knowledge of the farm, the geography, climate, soil, and history will all be relevant in deciding the correct approach for each specific area.
Case studies
Case study 1
The farm in case study 1 is an example of a farm where a change in farm management has been taken for economic reasons but also to ensure the farming business has a positive impact on the ground farmed (Figures 5 and 6). Within the space of 12 months the farm has converted from a 750 cow all-year-round calving indoor dairy to a 400 cow autumn block calving dairy with previously housed cows now grazing for 6 months of the year.
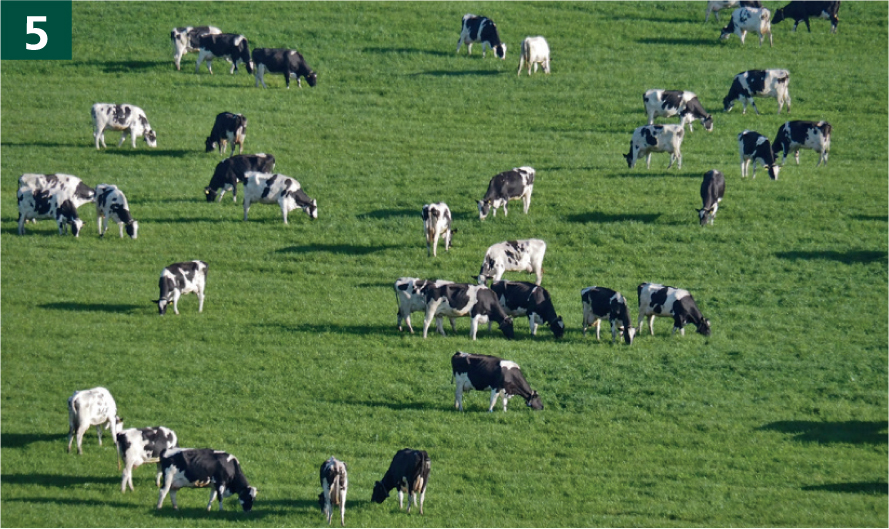
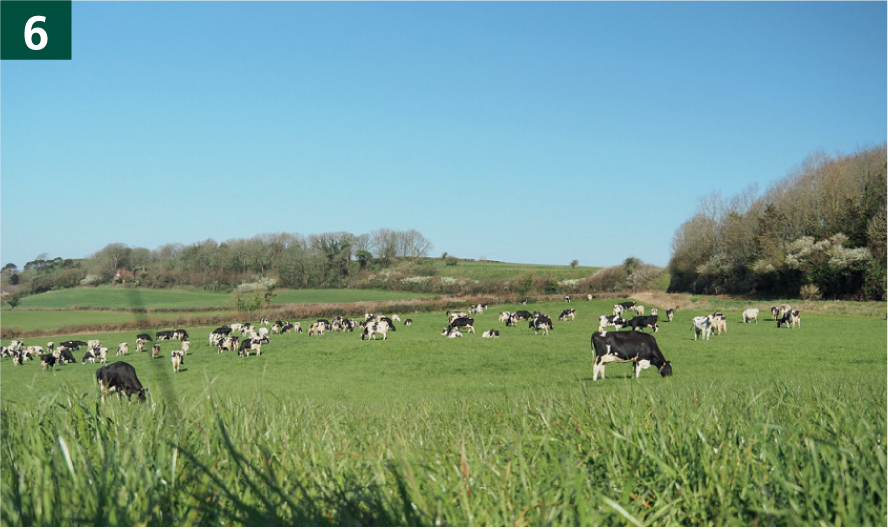
Farm facts
The farm is 809 ha (2000 acres) (Figure 7). It is now split into a maize, wheat and barley rotation (170 acres), a wheat and grass rotation and Countryside Stewardship areas. Previously 320 acres of maize were grown annually with mustard, brassicas and radish cover crops planted after harvest, which were grazed by sheep over winter. On previous silage ground, 220 acres of grazing has been established. Mixed herbal leys have been planted (a seed mixture of grasses, legumes and herbs). Clovers were utilised as a legume source in this mix and the chicory, sainfoin and birdsfoot trefoil were some of the herbs planted. In layperson's term, the grasses provide the carbohydrates (plus protein and lignin), the clovers add an increased protein component to the sward, and the forage herbs, for example chicory in this instance, offer both drought resistance and an ability to mine the soil for minerals because of their deep root structure. Chicory also contains natural anthelmintic properties (Nwafor et al, 2017). The high legume content of herbal leys reduces the need for artificial nitrogen, with a 40% reduction in artificial fertilizer in the first year after planting seen on this farm.
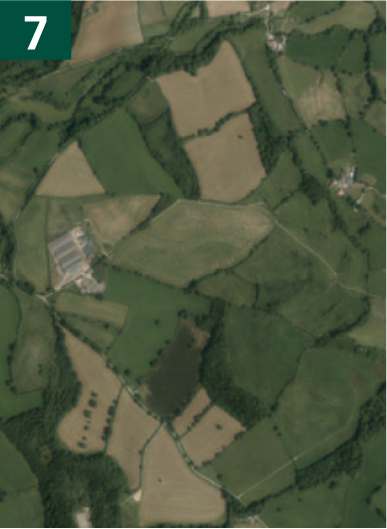
75 acres of winter bird cover is now planted annually and a further 75 acres of nectar mix is to remain in situ for 5 years, only to be grazed after late spring. Further ground has been left fallow for 2 years, with a legume mix planted to encourage both mammalian and insect biodiversity but also improve soil nitrogen content.
As part of Conservation Stewardship a further 35 acres of woodland buffer and river margins have been allowed to grow unchecked. From an animal husbandry perspective, fencing the river margins reduces the access of cattle to the intermediate stage of the liver fluke lifecycle, through reducing contact with the molluscan intermediate host Galba truncatula and aids in the reduction of pasture fluke contamination. From an ecosystem perspective, the banks of waterways are prone to poaching, with cattle's instinctive behaviour to congregate near natural water sources. Fencing waterways off from livestock reduces both waterway pollution and soil compaction along the waterway margins.
Management changes have resulted in:
- 400 Holsteins, down from 750 Holsteins
- Milked twice a day reduced from 3 times a day
- Average yield 30 litres a day, down from 38 litres a day
- Moving from all year round calving to autumn block calving
- 65–70% forage in ration, up from 45–50% forage
- Grazed outside from March until September.
Milk supplied to Arla. Previously over 10 million litres of milk were produced annually. This has now been reduced to 4.4 million litres. The farm owners were aware that their business prior to a change in management was no longer economically viable nor were they content with the affects on the local environment. By making the alterations the farm enterprise immediately returned to profit, and will be able to continue to dairy farm for successive generations to come. While the farm currently only produces 44% of previous milk yields, it will be able to produce that 44% for many years longer than the original model would have allowed. This example raises a salient point regarding future milk production within the UK. Wholescale adoption of lower input systems would result in production less than current market demands. It is an illustration of why the National Food Strategy favours a plethora of competing farming systems to feed the population in tandem with looking after the climate and environment.
Soil compaction
The annual physical compaction of the silage fields via farm machinery was having a cumulative detrimental effect on soil structure (Figure 8). The farm's soil had a large clay component. Clay soil's ability to store water and nutrients is in part because of the greater surface area of the smaller clay individual soil particles in comparison with sand, silt or loam soils. The same properties behind clay's benefits also present a challenge. The small size of clay particles, combined with their flat shape in comparison to round grains of sand allows for efficient stacking and compaction. This limits biological activity within the soil ecosystem, limiting plant root penetration and providing a significantly reduced area for mineral and nutrient exchange, reduced air spaces and reduced water storage capacity.
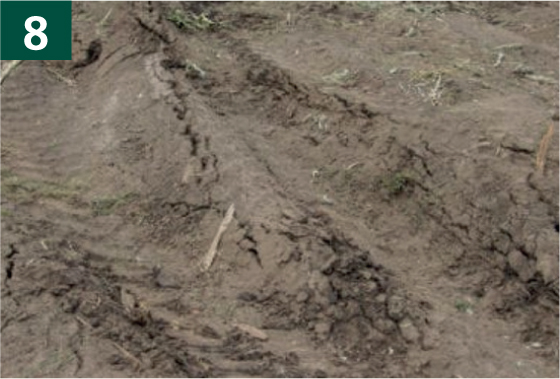
The aim has been to reduce machinery passage over the fields by conversion to a grazing system. However, there is a need to alter grazing management after the first summer. Walking the fields post grazing, it is evident that cow hooves have sunk 4 inches into the soil, and digging down there is a further 4–8 inches of compaction of the clay soil below. In year one 650 to 700 kg adult dairy cows were grazed on rotation at a moderate to high stocking density. Distributed over four hooves, each hoof might have 175 kgs pressing into the soil. As the cow walks, its full weight is distributed over only two or three hooves, resulting in 233–350 kgs over a few square centimetres of soil. The soil compaction we aimed to prevent from removing machinery has been replaced by grazing cattle. Stocking density, rotation length and paddock size all need to be considered for future years.
Figure 9 demonstrates deep compaction 50–100 mm deep, worsened by very wet conditions in spring 2021. Figure 10 shows shallower compaction likely caused by the impact of cows' feet in moderate to wet weather.
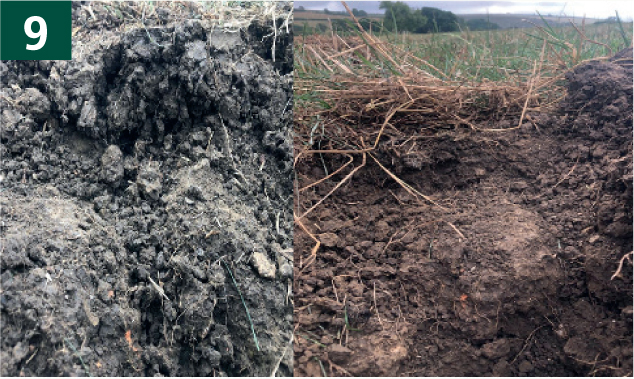
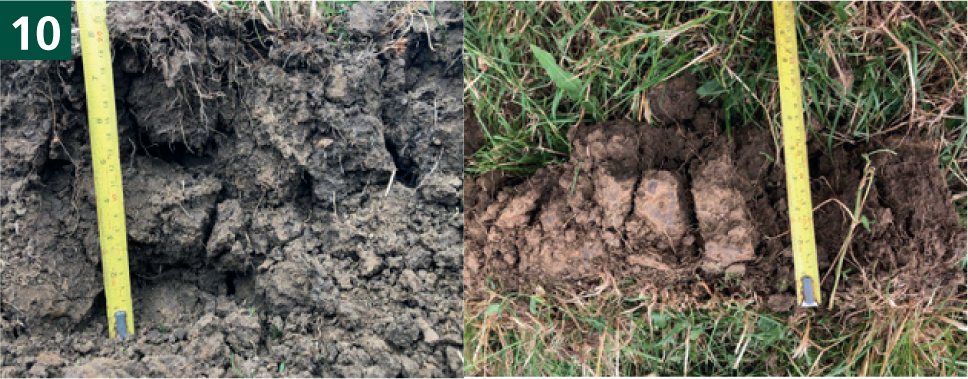
Case study 2
The farm in case study 2 was a West Dorset farm tenancy taken on by a inspirational couple entering the dairy industry in 2013 in a share farming agreement. Immediately an organic conversion was commenced with milk being sold to Arla from 2014 onwards.
The cows on farm:
- 360 cows split calving herd over 900 acres. Low input, low output system averaging 5500 litre 305 day milk yields, and the aim of the system is to maximise milk from grass and minimise bought in feeds
- 2018 started soil sampling and the declining quality of some soil parameters became quickly evident.
Mob grazing trial
The farm is now enrolled in a mob grazing trial alongside an innovative farmer's field trial. For this a 22 acre field has been divided 50/50 via the already existent cow track through the field's centre. Half the field is to be grazed conventionally (2800 kgs dry matter (DM) per hectare grazed down to 1500 kgs DM per hectare with small amounts of slurry application in between, while the other half is to be mob grazed with approximately a 60 day gap between grazings.
Pasture improvement
Having inherited perennial rye grass/white clover leys, and with the aim of creating diverse leys without having to plough and re-seed the fields (for economic as well as regenerative reasons) the farm has gradually converted the pasture. After the first 3 to 4 years, the white clover gradually began to die out, allowing for weed grasses to encroach the pasture. With the DIY creation of an ingenious spring tine harrow, the shallowing rooting weed grasses were able to be stripped out (removing 30–40% of the vegetation within each field) followed by the direct drilling of the chosen pasture mix. The mix was predominately plantain, sainfoin, white clover, and red clover within a rye grass ley. It is important to acknowledge the pasture mix for each field will vary according to which improvements in soil indices are being aimed for.
Compost project
With all-year-round grazing, there is never an ideal time to apply farmyard manure. Typically, this is done in the autumn once plant growth has slowed for the year to allow for it to decompose over winter. This has associated run-off and eutrophication concerns. By developing a composting system, the aim is to be able to provide a stable manure that can be applied to the growing swards at their time of requirement without environmental concerns. A Farming Protective Landscape grant has helped fund this trial.
Case study 3
The farm in case study 3 is an example of a large dairy cooperative's investigation into regenerative agriculture
Arla's regenerative farming pilot network
From September 2021, Arla has established a network of pilot farms to provide insights and learnings about how to support Arla's farmer owners in adopting more regenerative practices. There are 26 farms currently enrolled: four in Germany, two in Holland, six in Sweden, six in Denmark, and a final eight within the UK. The group comprises a mixture of organic, conventionally grazed and indoor intensive farms.
By collaborating closely with farmer owners, Arla wants farmers to drive the evolution and implementation of what it means to farm regeneratively in the context of dairy systems and make them an integral part of agreeing relevant principles and practices for success at scale. These pilots will also create an opportunity for members to meet on farms and gain inspiration, share experiences and learn from each other.
Conclusions
Veterinary surgeons are uniquely qualified to advise clients in how to operate in a climate and environmentally sensitive manner while continuing to work towards productivity and profitability. The practices incorporated within a regenerative agriculture approach offer potential to acheive this aim. After all, there is no Planet B.
KEY POINTS
- Agriculture needs to feed an ever-expanding global population while providing part of the solutions to both climate change and biodiversity loss.
- Economically, politically and morally no one wants the production of our inputs to the UK agricultural industry to be detrimental elsewhere in the world.
- Regenerative agriculture offers an approach with six practices at its core, rather than one approved definition.
- Regenerative agriculture aims to farm in harmony with nature, both above and below the ground, while simultaneously providing high quality food production and environmental stewardship.
- Regenerative practices are deemed currently to produce lower total outputs compared with conventional agricultural methods, however their lower cost of production model provides greater economic resilience and the potential for increased profitability.
- Soil health is at the core of the system. Global soil degradation rates are one of the greatest threats to current food production.