Ketosis causes infertility, loss of milk yield, and greater risk of other postpartum diseases. Veterinarians tend to be focused on ketones, perhaps because beta-hydroxybutyrate (BHB) is easy to measure, and acetone can be smelled on cows' breath. But ketones are not the cause of lost milk yield, and they are not a primary cause of infertility. Ketones might be a cause of the increased risk of other diseases: the mechanism for this is not known. When used without qualification, the word ‘ketosis’ herein refers both the clinical and the subclinical conditions.
Hyperketonaemia is a marker for the biochemical end stage of ketosis. However, the preceding stages are important in their own right. These stages are hypoglycaemia and a high concentration of free fatty acids (FFA, also known as non-esterified fatty acids, NEFA) in the blood.
Hypoglycaemia is important for two reasons. One is that suppression of hormone-sensitive lipase in adipose tissue by insulin is lifted, resulting in lipolysis, which releases glycerol and FFA. Hypoglycaemia is a primary driver of ketosis.
Secondly, hypoglycaemia is also the cause of infertility associated with ketosis as it reduces the cleavage rate of the fertilised oocyte. This effect is increased by a high concentration of BHB, but in the absence of hypoglycaemia, BHB does not affect the fertilised oocyte (Leroy et al, 2006).
FFA also cause infertility. They reduce fertilisation of oocytes, and their cleavage rate after fertilisation. Excess FFA are also the cause of reduced milk yield in cows with ketosis. They are useful as an energy source, notably in the mammary gland, undergoing beta-oxidation. But this produces reactive oxygen species (ROS), found to be present at a higher concentration in the mammary gland of cows with ketosis than in that of healthy cows (Song et al, 2021). ROS initiate apoptosis in the secretory cells. That apoptosis has two stages: a reversible stage followed by an irreversible stage. So, it is likely that prompt reduction in FFA concentration can protect the milk yield in the rest of the lactation.
What is the true prevalence of ketosis?
Hypoglycaemia, elevated FFA, and hyperketonaemia do not always occur together. A large survey in the UK found that 24.8% of lactating cows (all stages) had hyperketonaemia, a further 14.9% had elevated FFA concentration without hyperketonaemia, and 11.2% had hypoglycaemia without elevated FFA or hyperketonaemia (Macrae et al, 2019). If only BHB is measured in a herd, the extent of the problem will be grossly underestimated.
Is ketosis caused simply by negative energy balance?
Ketosis was previously thought to arise simply because the energy content of the cow's diet did not meet her requirements, and she was in negative energy balance. This caused hypoglycaemia which suppressed insulin secretion, allowing activation of hormone-sensitive lipase, and fatty acids were mobilised from triacylglycerol in adipose tissue. Beta-oxidation of FFA created an excess of acetyl-CoA in excess of the quantity that could react with oxaloacetate to enter the citric-acid cycle, and this excess was converted to ketones, including BHB.
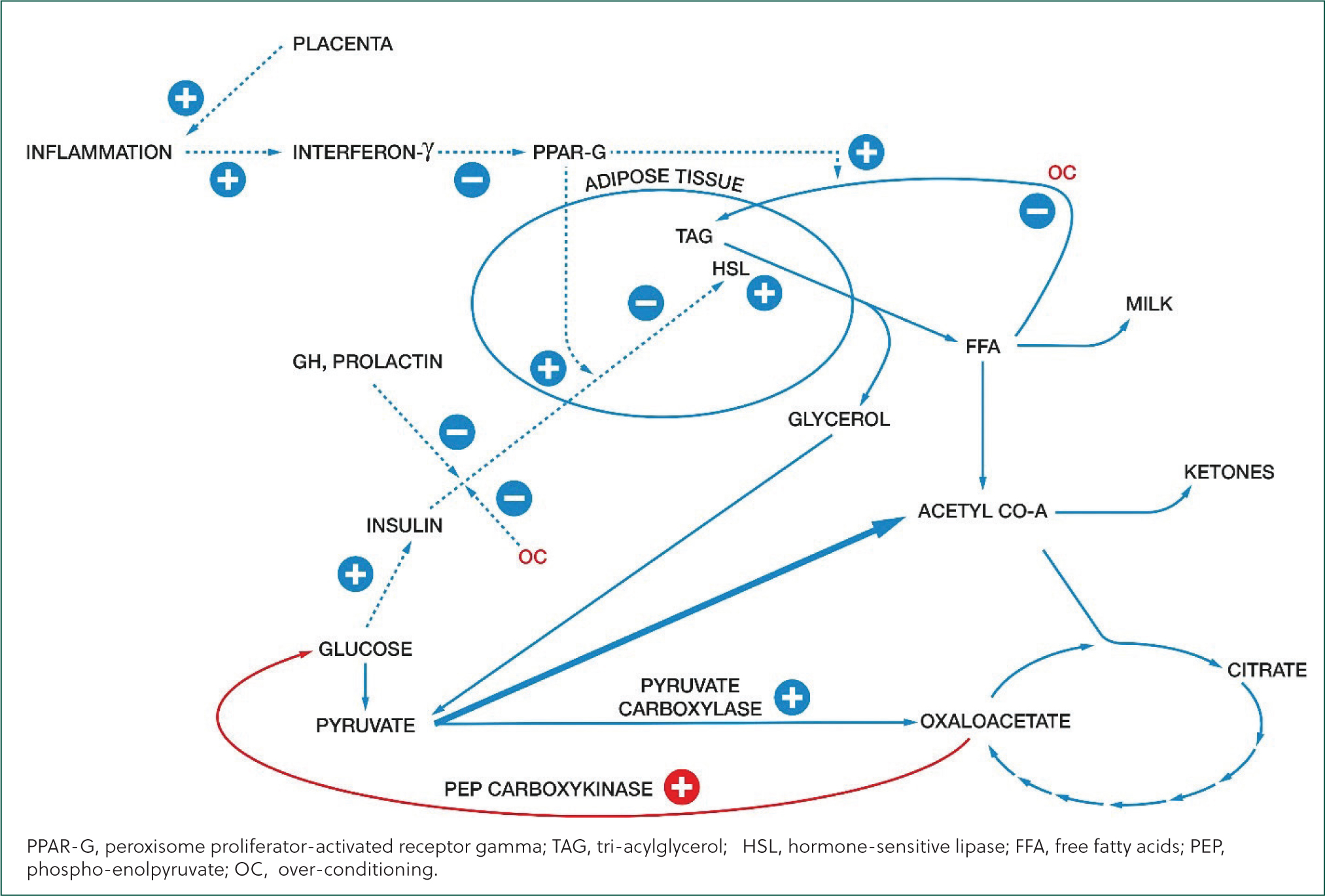
Box 1.Biochemical changes typical of the syndrome of ketosisThe syndrome of ketosis
- Hypoglycaemia
- Primary driver of ketosis
- Reduces cleavage rate in the fertilised oocyte
- High blood free fatty acid concentration
- Reduces fertilisation of the oocyte
- Reduces cleavage rate in the fertilised oocyte
- Reduces milk yield in two stages: reversible and irreversible
- Hyperketonaemia
- Potentiates the effect of hypoglycaemia on fertility
- Reduces appetite, further worsening the problem
In the early nineties, it was realized that insulin resistance was also a factor. This is the phenomenon by which the responses to insulin are reduced or cancelled, increasing fatty-acid mobilisation. Insulin resistance increases the concentration of FFA in the circulation, not just by increasing lipolysis but also by reducing lipogenesis, including re-esterification in adipose cells of FFA mobilised from adipose tissue. Insulin resistance is driven by the hormonal changes at the end of pregnancy and the start of lactation. It fulfils the useful function of increasing the availability of fatty acids for milk production and is mediated through effects on the expression of various genes. Cows that develop hyperketonaemia and hyperlipidaemia have a dysfunctional excess of insulin resistance, which exacerbates negative energy balance to create a pathological state.
Why does excess insulin resistance arise?
Insulin resistance has been inadvertently genetically selected. In a pair of recent studies, it was found that breeding value for milk production was a significant factor in reduced mRNA abundance, in subcutaneous adipose tissue, of three relevant genes coding for sensitivity to insulin. Overall, the higher the genetic merit for milk production, the lower the expression of these genes (Jaakson et al, 2018; Karis et al, 2020).
The other factor in insulin resistance is inflammation. Recent studies have shown that certain changes of behaviour and blood biochemistry before calving are associated with ketosis postpartum. In cows wearing an accelerometer on a hind leg, it can be seen that standing time in individuals that go on to develop ketosis is significantly less in the 20 days before calving onwards than it is in those that remain healthy (Rodriguez-Jimenez et al, 2018).
These findings led Mezzetti et al (2019) to investigate the hypothesis that cows which develop sub-clinical ketosis after calving have immune dysfunction during the dry period. They found that the concentrations of pro-inflammatory cytokines IL-1β and IL-6 were found to be increased at least 28 days before calving in cows that developed sub-clinical ketosis, with blood BHB concentration greater than 1.4 mmol/l, than they were in cows that remained healthy, indicating activation of the immune system before calving.
They found numerous similarities between dry cows that went on to develop sub-clinical ketosis and women who develop pre-eclampsia in pregnancy. This is a condition associated with restricted development of the placental circulation, placental ischaemia and oxidative stress. It causes a systemic inflammatory response similar to the inflammatory state of cows that go on to develop ketosis. This led Mettezzi et al (2019) to speculate that a subclinical dysfunction of the placenta may cause ketosis.
One well-defined effect of inflammation concerns peroxisome proliferator-activated receptor gamma (PPAR-G). This is a receptor present in adipose tissue and has a major effect on maintenance of insulin sensitivity. Inflammation is marked by production of interferon-gamma, a pro-inflammatory cytokine that suppresses PPAR-G production. Cows that are going to develop ketosis exhibit increased interferon-gamma release from artificially stimulated leukocytes three weeks before calving. The effect of this is to increase insulin resistance. The difference in interferon-gamma production between cows that will develop ketosis and those that do not is large, with a small standard error (Mezzetti et al, 2019). That suggests that, in modern, highly productive cows, inflammation is always a precursor to ketosis.
Other genetic factors
New genetic factors affecting the risk of ketosis are being found with the application of molecular techniques. One of these is particularly interesting because it explains why some cows have a high plasma concentration of FFA without a high concentration of BHB. Cows with relatively strong expression of pyruvate carboxylase will synthesize more oxaloacetate from pyruvate than others. This improves the availability of oxaloacetate to allow the entry of acetyl-CoA to the citric-acid cycle. So, despite the high concentration of FFA, there is no surplus of acetyl-CoA for conversion to ketones.
On the other hand, cows with relatively strong expression of phospho-enol-pyruvate carboxylase will draw more oxaloacetate from the citric-acid cycle, for gluconeogenesis, than others. In these individuals, the inadequacy of oxaloacetate for entry of acetyl-CoA to the citric-acid cycle causes its conversion to ketones.
The ratio of expression of these genes has been shown to be a major determinant of ketosis (Weld et al, 2020).
Selecting replacements
Since FFA inhibit the maturation and development of the oocyst, it is to be expected that dairy cows with better fertility have a lower periparturient blood FFA concentration. Furthermore, at low plasma glucose concentration, BHB also inhibits the development of the oocyst, so one would expect cows with better fertility to be cows with lower concentrations of BHB and higher concentrations of glucose.
We now have genomic tools to investigate this. Daughter pregnancy rate expresses a sire's genetic merit for the proportion of his daughters that become pregnant within 21 days of the voluntary wait period compared with a reference population. It can be predicted from a number of single-nucleotide polymorphisms, and this applies not only to sires but also to dams. Higher genomic merit for daughter pregnancy rate (GDPR) is associated with improvements in various indices of fertility (Lima et al, 2020).
Chebel and Veronese (2020) genotyped 821 heifer calves using Zoetis's Clarifide platform. They used the results to classify them into four quartiles of genomic merit for daughter pregnancy rate (GDPR): quartile 1 had the lowest merit and quartile 4 had the highest.
The ranking of these quartiles for GDPR matched their ranking for postpartum IGF-1 concentration, a hormone which increases insulin sensitivity and stimulates follicular growth, and blood glucose concentration. It inversely matched their ranking for post-partum FFA concentration and BHB concentration. It also inversely matched the incidence of ketosis.
In a herd wishing to reduce rates of clinical and subclinical ketosis and improve early lactation fertility, selecting replacement heifers with high genetic merit for daughter pregnancy rate can clearly reduce the unfavourable effects of high FFA concentration, and hyperketonaemia in hypoglycaemic cows, on fertility.
Anti-inflammatories
Historically practitioners have administered a single dose of corticosteroids (dexamethasone) to hyperketonaemic cows. However, recent evidence has demonstrated that this is seldom indicated, with benefits only being observed in the mildest of cases. Tatone et al (2016) found that a single intramuscular injection of dexamethasone alongside treatment with propylene glycol only reduced serum BHB concentrations if they were initially between 1.2 and 1.5 mmol/L. Tatone et al (2016) also demonstrated that administering dexamethasone to cases where serum BHB concentrations exceed 3.2 mmol/L increased the likelihood that the cow would remain hyperketonaemic 14 days later.
Since inflammation plays an important role in the development of ketosis, through increasing insulin resistance, anti-inflammatories may prevent it. Kovacevic et al (2019) demonstrated that this is the case. Study cows were given 3 mg of ketoprofen per kg liveweight by intramuscular injection daily for three days after calving, or an equal volume of saline. Eight and 15 days after calving, blood concentrations of TNF-α, IL-1α and IFN-γ were substantially lower in the ketoprofen-treated cows. They also had lower FFA and BHB concentrations.
The use of anti-inflammatories after calving is advocated after assisted or extended delivery. This study suggests there may also be a place for it in cows judged from their condition score or disease history to be most at risk of excess lipolysis.
Treating ketosis
Propylene glycol is a well-established treatment for ketosis, given as once- or twice-daily doses over three or four days. The dose is limited by its toxicity, which is apparent especially at more than 500 g per day (Neilsen and Invartsen, 2004).
Studies of its effect on plasma concentration have given radically conflicting results. Some have shown that it raises blood glucose concentration within an hour. Others have shown that it does not do so for eight hours (Pethick et al, 1999; Alon, 2020).
There has been only one substantial study of its effect on plasma BHB concentration, performed in cows with a starting concentration of BHB between 1.2–2.9 mmol per litre. In total, 80% of the treated cows returned to normal serum BHB concentration after nine doses (McArt et al, 2011).
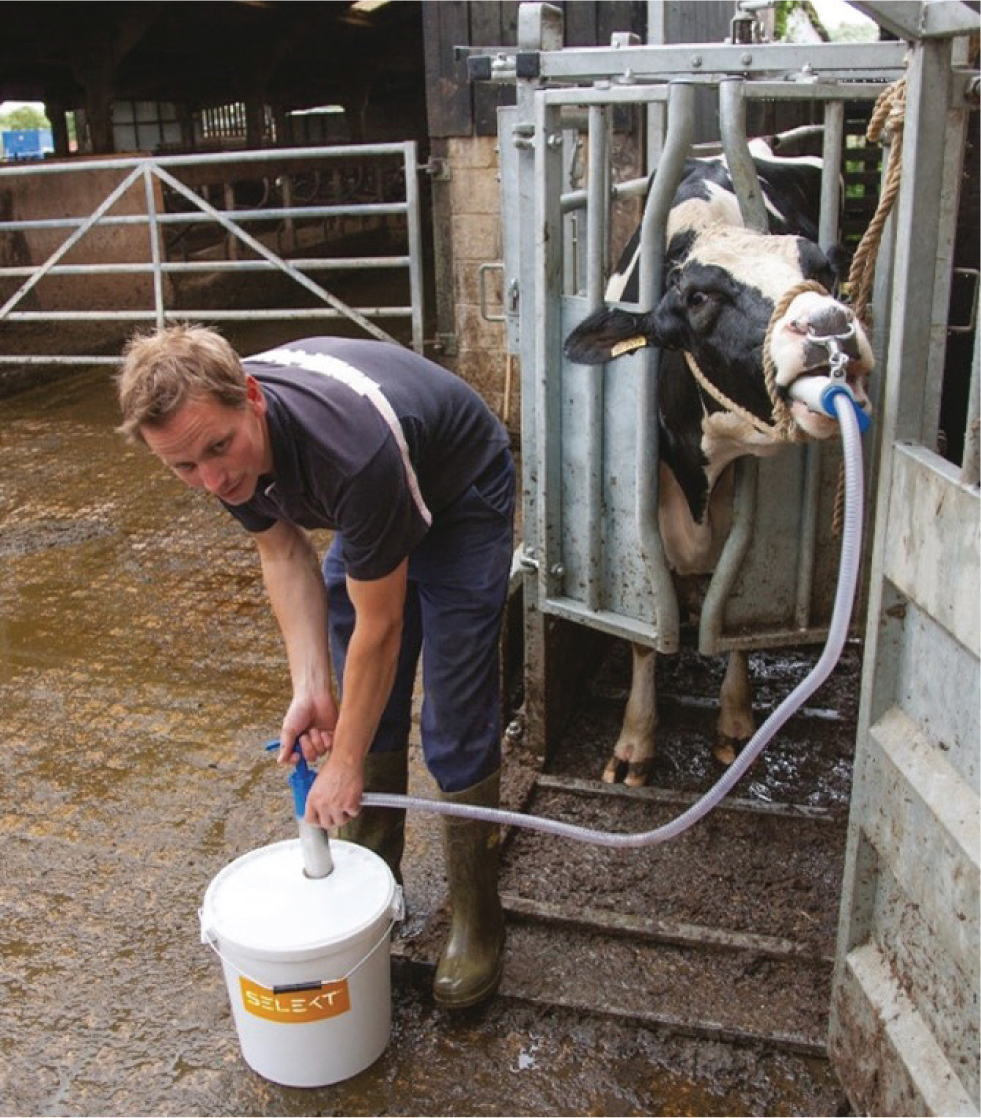
Glycerol is an alternative treatment, first studied in 1954 when Johnson reported a clinical cure of a refractory case with a single dose of 2.25 kg. Goff and Horst (2003) reported two clinical cures with a single dose of 1 litre, noting also that they found 3 litres to be toxic. Like propylene glycol, glycerol has given conflicting results in respect of blood glucose concentration.
A study by Alon et al (2020) in ewes 132 days into pregnancy, carrying, on average, more than two foeti, is instructive in showing the effects of propylene glycol and glycerol. Blood glucose concentration was raised substantially by glycerol within an hour. In the group given propylene glycol, it did not exceed the concentration in a group given water as a control for 9 hours. Various studies of the metabolism of propylene glycol and glycerol suggest that this is explained by the shortness of the metabolic pathway for conversion of glycerol to glucose, and the greater length of the pathway for conversion of propylene glycol to glucose.]
Alon et al (2020) also found that propylene glycol rapidly and substantially reduced blood BHB concentration. That is explained by the short pathway for its conversion to oxaloacetate. By providing this, propylene glycol allows acetyl-CoA entry into the citric-acid cycle, rather than leaving it for conversion to ketones.
Glycerol has a slower and lesser effect on BHB concentration. The pathway for its conversion to oxaloacetate is long; it probably exerts its effect on BHB by reducing fat mobilisation as it increases blood glucose and insulin concentration.
Both propylene glycol and glycerol reduce blood FFA concentration. Propylene glycol does so more quickly. That is explained by its initial conversion to lactate, which serves as a messenger in the signal by which insulin reduces lipolysis. The effect of glycerol here must be a consequence of its raising blood glucose concentration.
Finally, the study of Alon et al (2020) sheds light on the inconsistent effects of propylene glycol on blood glucose concentration. It differs from studies which have found a rapid effect because it was done in ewes with a relatively high BHB concentration. This suggests that the first priority for metabolism of the oxaloacetate it yields is anaplerosis (supplying the citric-acid cycle). When it is not needed for anaplerosis, it can be used for gluocogenesis.
Another informative study by Hippen et al (2008) compared the effects of glycerol given by various means, including pump-drenching. They concluded that ‘to be glucogenic, glycerol must either be delivered with water to associate with the liquid fraction of the rumen contents, or be able to “bypass” the rumen in some form to be absorbed as glycerol and converted to glucose by the liver.’ This is probably because the water reduces the viscosity of the glycerol. Undiluted glycerol is viscous, so it will permeate the rumen mat only slowly, allowing time for its fermentation. But water has a powerful effect on its viscosity. A mixture which includes 15% water has a viscosity only 15% that of pure glycerol. It will penetrate the rumen mat quickly, allowing it to be absorbed unchanged. Studies in which glycerol has been found to raise blood glucose concentration quickly, including that of Alon et al (2020), were done with glycerol mixed with water.
The authors' company, taking account of these findings, manufactures a mixture containing 630 g of glycerol and 320 g of propylene glycol. It is called Selekt Glycerol Plus, and it is recommended that the full quantity be given with water to clinical cases. It has been observed that this one intervention resolves the signs of ketosis. A study has found that it needs to be given twice or three times to restore BHB concentration to normal in cases of clinical ketosis (Wood, 2014). This compares favourably with the nine doses found to be required for the same result in sub-clinical cases in McArt's study of 2011.
For efficacy, Selekt Glycerol Plus must be given with water. That is commonly indicated in any case. Ketosis is often described as a dehydrating condition. This is for several reasons, the most important of which is that ketones are osmotic diuretics. In man, Ooi et al (1997) found that log of plasma BHB concentration was the strongest predictor of dehydration amongst a range of biochemical variables. They went on to propose the use of urine ketones as an indicator of dehydration.
Conclusions
It has been known for a long time that hypoglycaemia is an important factor in infertility. It is now known that the harm caused by hyperketonaemia for fertility depends on hypoglycaemia. The importance of excess FFA has also been shown. Pump-drenching a ketotic cow with Selekt Glycerol Plus in water will correct all the abnormalities present, restoring hydration and blood glucose concentration, and reducing BHB and FFA. This means it can resolve the clinical signs, improve milk yield, fertility, and hydration, and reduce the risk of secondary disease.
KEY POINTS
- Hypoglycaemia is a primary driver of ketosis.
- Hypoglycaemia and excess free fatty acids are the causes of the infertility associated with ketosis.
- Recent studies have shown that only measuring serum beta-hydroxybutyrate concentrations may understimate the prevalence of ketosis in the herd.
- Selecting replacements with high genetic merit for daughter pregnancy rate can reduce the unfavourable effects of hyperketonaemia and high free fatty acids (FFA) on fertility.
- Providing ketotic cows with a product containing glycerol and propylene glycol in water will restore hydration and blood glucose concentration. It will also reduce blood BHB and FFA.