Iron-deficiency anaemia is a well-established condition in neonatal piglets. It has been identified in calves and lambs for a long time, but more recent research in several countries has led to an increasing awareness of the condition in those species. This article gives an overview of the current knowledge.
Iron in the body and its regulation
Most of the iron in the body is stored in haemoglobin (60–70%), while iron can also be found in haemosiderin (spleen, liver) and myoglobin. Some is transported in blood, bound to transferrin. Apart from its function in transporting oxygen in haemoglobin and storing oxygen in myoglobin, it is an essential part of enzymes such as peroxidases, catalases and cytochromes and also works as an antioxidant. Therefore, iron deficiency is not only associated with anaemia but also with oxidative stress (Rajabian et al, 2017) and a reduced immune response (Gygax et al, 1993). In piglets, iron has been shown to directly promote intestinal development (Pu et al, 2018), brain development (Leyshon et al, 2016) and spacial cognition (Rytych et al, 2012).
Iron homoeostasis is tightly regulated, with hepcidin (a peptide hormone produced in the liver) playing a central role. If the iron concentration in blood increases, or in the presence of inflammatory mediators, hepcidin downregulates intestinal absorption and leads to more intracellular storage of iron in the reticuloendothelial system, monocytes and macrophages. This is important for two reasons:
- Excessive free iron and haem (in haemolytic situations) can generate toxic free radicals, resulting in tissue damage
- Iron is also a nutrient for pathogens, and limitation of iron is a well-established mechanism to control infections. In another context, this strategy is well known in dry cows producing lactoferrin in the udder.
A unique feature of iron is that its regulation (via hepcidin) is not only influenced by its concentration but also by the host's infection status – hepcidin gene expression is upregulated in the presence of pathogens and cytokines, limiting the iron supply to bacteria and protozoa and also reducing the potential for viruses to replicate (Drakesmith and Prentice, 2012).
Iron content of whole milk and iron demand
Neonatal piglets, calves, lambs and kids are born with a finite iron store, leading to a rapid decline in haemoglobin levels in the first 1–2 weeks. Cows' milk contains around 0.5 mg of iron per kg (Ziegler, 2011), while the iron demand of a 6-week-old pre-weaned dairy calf is stated to be around 135 mg (National Research Council, 2001). It is obvious that whole milk cannot supply enough iron to meet the demand, and with more recent recommendations to increase the intensity of milk feeding and later weaning, this discrepancy may be exacerbated. Almost all commercial milk replacers are therefore supplemented with around 100 mg/kg dry matter of iron. A similar discrepancy is known in piglets and lambs. However, outdoor reared pigs root in the soil from an early age, which contains high amounts of iron, so the problem is more prominent in indoor reared pigs. Iron supplementation has been shown to be beneficial in outdoor reared piglets as well (Szabo and Bilkei, 2002) but is practically more difficult.
Iron-deficiency anaemia shows haematologically as a microcytotic, hypochromic anaemia (Joerling and Doll, 2019).
Definitions and symptoms of subclinical iron-deficiency anaemia in calves and lambs and diagnostic tools
While clinical anaemia is readily diagnosed following a physical examination, subclinical anaemia often remains unnoticed. Reduced growth rates have been established in calves (Allan et al, 2020) and lambs (Crilly and Plate, 2022) in intervention studies. A higher frequency of neonatal disease like diarrhoea or pneumonia in calves (Bünger et al, 1986) and a higher frequency of abomasal bloat in lambs has also been described (Vatn and Torsteinbø, 2000). In contrast, Lorenz et al (2021) found that iron supplementation at birth was a risk factor for neonatal diarrhoea in calves, but did not distinguish between oral and parenteral supplementation. This apparent paradox is discussed below.
While serum iron concentration and ferritin levels have been discussed as potential parameters to evaluate the iron status, both have methodological difficulties. Serum iron levels show considerable circadian variation and are also affected by inflammatory processes (it is also part of haptoglobin), while ferritin is species specific and therefore not available in routine veterinary diagnostics. The correlation between serum iron levels and haemoglobin concentration is weak (r=0.35; Doll, 2018). Haemoglobin concentration is most frequently used to define anaemia, although its suitability has also been questioned (Joerling and Doll, 2019). It is the basis of most animal welfare legislation, such as that regarding veal calves. However, there is no generally agreed reference range for haemoglobin levels in calves, with different authors and sources stating different lower levels to define anaemia. EU and UK law (Welfare of Farmed Animals Regulations 2007) and the UK Welfare Code (Department for Environment, Food and Rural Affairs, 2013) both require diets leading to a minimum of 4.5 mmol/litre haemoglobin, while German regulation (as a group average) and the Royal Society for the Prevention of Cruelty to Animals (2020) accreditation require 6 mmol/litre (Bundesministerium der Justiz, 2021).
Prevalence of iron-deficiency anaemia
Calves
Doll (2018) surveyed 128 calves on 23 German farms, of which 18 were dairy farms and five were beef suckler herds, and found the majority of dairy calves (sampled up to 3 months of age) to be anaemic when using the cut-off point of 6 mmol/litre haemoglobin (Figure 1). Only seven of the 18 dairy farms surveyed fed their calves on whole milk, and only one dairy farm gave oral iron supplementation at birth.
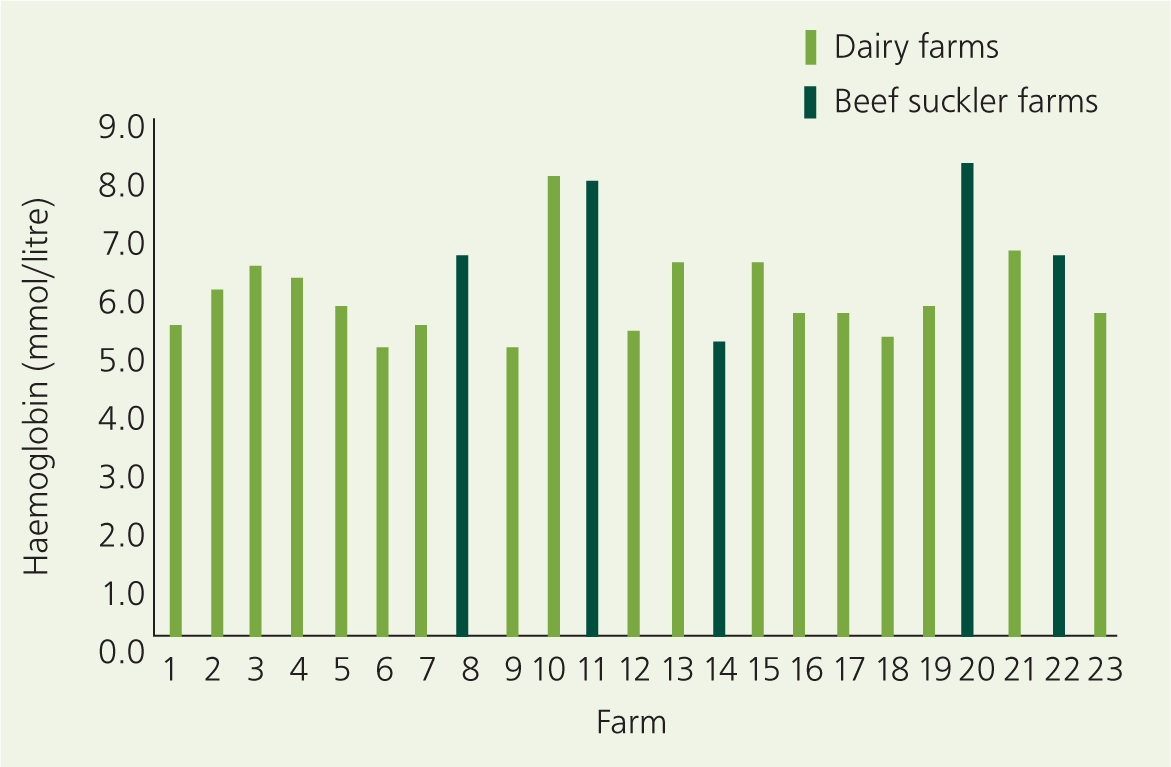
In a separate German study, Joerling and Doll (2019) found eight out of 40 calves (20%) were anaemic, under different feeding regimens, using a cut-off point of 5.6 mmol/litre. In the UK, Allan et al (2020) found that 40 out of 117 untreated dairy calves (34 %), all fed on whole milk, had haemoglobin levels of under 5.6 mmol/litre (9 g/dl) at around 6 weeks of age.
Lambs
Green et al (1997) found 25% of untreated lambs had a haemoglobin concentration below 8.7 g/dl (5.4 mmol/litre). Crilly and Plate (2022) looked at haemoglobin levels in lambs and goat kids under different feeding and management strategies and found 45% of goat kids and 80% of lambs were anaemic (haemoglobin below 8 g/dl; 5 mmol/litre) when reared indoors on maternal milk, while outdoor and/or milk replacer fed animals showed a lower percentage of anaemia (Table 1).
Table 1. Mean haemoglobin levels and % animals below 8 mg/dl haemoglobin of lambs and kids reared under different feeding and management strategies (each group contains 20 animals)
Group | Species | Environment | Diet | Mean [haemoglobin] (g/dl) | 95% confidence interval | % [haemoglobin] <ref range |
---|---|---|---|---|---|---|
1 | Goat | Indoors | Milk replacer | 9.65 | 9.07–10.23 | 5 |
2 | Goat | Indoors | Suckling | 7.73 | 7.21–8.24 | 45 |
3 | Sheep | Indoors | Milk replacer | 10.13 | 9.37–10.89 | 5 |
4 | Sheep | Indoors | Suckling | 6.8 | 6.14–7.46 | 80 |
5 | Sheep | Outdoors | Suckling | 9.08 | 8.49–9.66 | 20 |
Effects of iron supplementation
Calves
Iron-deficiency anaemia in calves and supplementation trials have been described since the middle of the 20th century (Hibbs et al, 1963). Bünger et al (1986) carried out extensive research in the former East Germany in the 1980s. In one study, 195 male calves from four source farms on the rearing unit were divided into three groups – no iron substitution, daily oral and two intramuscular injections of parenteral iron substitution given 33 days apart. Calves were sourced and treated from about 3 weeks of age, and the trial lasted 8 weeks. Iron-substituted calves had significantly lower treatment rates for both diarrhoea and pneumonia. The authors point out that the effect on disease rates was observed before the anaemia was corrected, suggesting a direct immunological effect of iron.
Heidarpour Bami et al (2008) supplemented neonatal dairy calves in Iran with 1000 mg iron as iron dextrane parenterally and found 40 g difference in daily liveweight gain (320 v 280 g) in supplemented calves, but no effects on calf health.
Allan et al (2020) carried out a supplementation trial on six UK dairy farms feeding whole milk. Of 237 enrolled calves, 120 were injected with 1000 mg iron as iron dextrane on days 2–10. Between enrolment and 6 weeks, injected calves grew on average 78 g per day faster than control calves. The effect did not continue from 6–12 weeks, so although the 0–12-week daily liveweight gain was significantly different, this is purely the result of the difference in the first 6 weeks. Haemoglobin levels were measured at enrolment and at 6 weeks – control calves dropped on average by 12.1 g/litre while supplemented ones gained on average 3.4 g/litre, making the difference at 6 weeks 15.3 g/litre. A total of 40 animals in the control group and six in the supplemented group were considered anaemic at 6 weeks (haemoglobin under 90 g/litre). Health data were not collected in this study. The growth response to iron supplementation on individual farms in this study was not linked to the haemoglobin concentrations of untreated animals, so although the effect was consistent between farms its magnitude cannot currently be predicted.
In contrast to earlier studies, Lorenz et al (2021) investigated risk factors for neonatal diarrhoea on Bavarian dairy farms and found ‘iron supplementation after birth’ was a significant factor. They compared 59 farms with a diarrhoea problem with 18 control farms, so this was a retrospective analysis, not an intervention trial, and the exact timing and method of iron supplementation (oral vs parenteral) was not stated on the farms. While there is the possibility that problem farms were more likely to supplement, other preventive measures were not carried out at higher frequency. The authors discussed the possibility that in some circumstances, iron may favour pathogens, as iron depletion (for example, via intra-cellular storage, triggered by hepcidin) is a recognised pathway of defence against pathogens (Drakesmith and Prentice, 2012). However, they stated that further research is needed to validate the advantages and disadvantages of iron supplementation, and different timings and means of administration.
Lambs
Green et al (1997) found that housed lambs treated with 300 mg iron as iron dextran were heavier (1 kg) at weaning than untreated controls, with a trend to lower mortality. Haematological parameters at 12 and 24 days of age were also significantly improved. In Norway, Vatn and Torsteinbø (2000) found reduced serum iron concentrations in housed lambs that developed abomasal bloat, compared to those who did not develop the condition, and subsequently significantly reduced the incidence by supplementing iron (300 mg of iron as iron dextrane subcutaneously in the first week of life). Supplemented animals also had a daily liveweight gain in-crease by 17 g in the first spring and an overall average liveweight gain increase of 7 g per day from birth to slaughter.
Crilly and Plate (2022) also found increased liveweight gains in treated lambs in the UK, but the difference to control animals was only significant for twin lambs, the overall difference here was 23 g per day. Again, a significant difference in haemoglobin levels was observed at 1 month of age.
Conclusions
Iron-deficiency anaemia is common in housed calves and lambs fed on whole milk, and studies on the effects of iron supplementation on growth rates and haemoglobin levels favour supplementation. Some studies have shown health benefits (reduced incidence of pneumonia and diarrhoea in calves, reduced incidence of abomasal bloat in lambs), but others have not found any health or mortality effect, and one retrospective analysis of risk factors for neonatal calf diarrhoea questions the usefulness of iron supplementation. Further studies are needed to establish optimum timing and means of administration.
In practice, iron-deficiency anaemia should be considered as a risk factor when investigating the conditions described, and haemoglobin levels at 2–6 weeks of age may indicate the severity of a problem. Unlike in other European countries, there is no licensed parenteral iron injection for calves and lambs in the UK, so if this route of administration is chosen, any treatment will have to be given off label, using a preparation licensed for pigs. The recent literature is consistent in using 1000 mg iron for calves and 300 mg for lambs once, soon after birth, so these doses can be recommended. Increased iron supplementation of dams during pregnancy has not shown any significant effects in pigs or cattle.
KEY POINTS
- Iron deficiency anaemia is a prevalent and often underdiagnosed condition in housed calves and lambs fed on whole milk.
- Clinical signs are reduced growth rates and potentially increased risk of infectious diseases.
- Monitoring via haemoglobin screening should be carried out in potential problem herds or flocks.
- Supplementation of iron should be considered in problem herds or flocks.