All manufacturers of new and existing veterinary medicines have to provide a satisfactory environmental risk assessment (ERA) to the regulatory authorities in order to register a new product or renew a marketing approval (MA). There is a strong focus on products that have insecticidal properties and that are used in farm livestock because of their potential impact in both aquatic and terrestrial ecosystems (Liebig et al, 2010). ERAs are generated within a framework that incorporates the physicochemistry, pharmacokinetics and toxicology of compounds and tiered testing that ranges from short-term, single species toxicity evaluations to long-term, complex ecological studies within agricultural landscapes (Wratten and Forbes, 1996). The rationale behind the tiered approach is to generate data through studies of increasing complexity, which will eventually lead to an objective, quantitative assessment of risk in agricultural landscapes; components of such testing regimens include:
- Hazard identification
- Physicochemical profile
- Pharmacokinetics, metabolism and excretion patterns
- Hazard characterisation
- Nature of biological effects
- Dose relationships
- Degradation and disappearance of compound in the environment
- Exposure characterisation
- Concentrations of compounds (and metabolites) in water, sediments, soil and faeces over time
- Phenology (seasonal activity and biology) of susceptible organisms and their presence in contaminated substrates
- Frequency and duration of contact between organism and residue
- Magnitude of refugia
- Risk characterisation
- Quantitative estimation of risk
- Ecological models
- Large-scale, long-term field studies.
Though most insecticides are potentially hazardous to aquatic invertebrates, under practical livestock farming conditions, the greatest threat comes from the incorrect/illegal disposal of sheep dips and run-off from parasiticide sprays (Beynon, 2012), leading to contamination of water courses (Rasmussen et al, 2013; Antwi and Reddy, 2015). Water quality is subject to routine monitoring by various local and national authorities and penalties can be issued if contamination from agricultural sources does occur and veterinary clinicians should be aware of the risks and provide advice when applicable. However, the subject that farm animal clinicians are most likely to encounter in practice is the potential environmental impact of veterinary products with insecticidal properties on the insect fauna of (cattle) dung.
Hundreds of papers have been published on the subject, including numerous reviews; a special issue of Veterinary Parasitology, Environmental impact of avermectin usage in livestock (Volume 48, pages 1–343) was published in 1993 following a multinational 3-day conference and the subject has been covered extensively in several textbooks (Roncalli, 1989; Steel and Wardhaugh, 2002; Scholtz et al, 2009) and in popular science (Jones, 2017). That is not to say that the scientific literature provides adequate or balanced coverage of the subject as it is heavily biased towards the macrocyclic lactones (MLs), particularly ivermectin, which has become the ‘standard’ for basic research and comparative studies. Inevitably therefore, much of the material for this article focuses on MLs, if for no other reason than data for other compounds simply do not yet exist, however a short section is devoted to the synthetic pyrethroids (SPs) and triclabendazole.
Veterinary parasiticides and dung insect fauna
Although the potential for insecticides to affect non-target dung fauna and function had been recognised for some time (Anderson, 1966; Miller and Pickens, 1973; Schmidt, 1983; Anderson et al, 1984), it was not until the late 1980s that potential environmental impact of veterinary medicines first caught the public eye with an article that appeared in the journal Nature, which described some observations on the effect of a prototype, long-acting-bolus formulation of ivermectin on coprophagous insect larvae and the degradation of dung pats from treated cattle (Wall and Strong, 1987). Despite several methodological short-comings in this study, most notably a lack of adequate replication (Wall and Lee, 2010a), the conclusions, though highly speculative, were quickly assumed to be valid by many scientists, conservationists and the media and this remains the case to this day. The two principal predictions raised by the authors were:
- ‘Increasing use of ivermectin in cattle is likely to pose a serious ecological threat to those insects (particularly Diptera spp.) that have evolved to exist uniquely in conjunction with cattle dung’
- ‘Retarding pat decomposition may increase the amount of pasture fouled by cattle dung and, therefore, could have important consequences for grassland husbandry’.
The purpose of this article is to provide a brief summary of developments over the >30 years since these initial results were published, with emphasis on what is applicable to the UK and Ireland. Before examining the applied literature, a brief synopsis of the natural biology of dung fauna and flora and the processes involved in the disintegration of dung in temperate regions is provided to give context to any natural or unnatural perturbations.
Cattle dung and its invertebrate fauna and flora
Cattle dung is an aqueous matrix of undigested herbage cellulose and lignin, minerals, dead or living microorganisms, cellular debris, mucus and metabolites. Various pathogenic viruses, bacteria and parasites are also commonly passed out of the host in the excreta. The quantity and composition of dung produced by cattle varies considerably and is affected by several factors, including feed intake, diet, liveweight, physiological status, parasitism and health. A 600 kg cow typically produces around 30 kg fresh faeces, equivalent to ~5% of live weight (LW), per day; the average number of defecations per day for cows is 11–12, indicating that each pat is of around 2.5 kg fresh weight (Marsh and Campling, 1970). Faeces are passed in a non-random manner by free-ranging cattle and the pattern of deposition on pasture follows a negative binomial distribution function, with concentrations of pats at various sites including resting, watering areas and other locations where cattle congregate (Petersen et al, 1956).
The dung invertebrate fauna comprise a complex of arthropods, including beetles, flies and mites, as well as annelids, nematodes and microorganisms such as bacteria and fungi (Skidmore, 1991); this community includes not only obligatory and facultative coprophages, but also saprophages, fungivores, predators and parasitoids. In the UK, over 250 insect species are associated with cow dung (Skidmore, 1991). The relative occurrence and abundance of individual species within cow dung depends on several factors including the geographical location, the age of the pat, its site of deposition, the season of the year and the prevailing weather.
Arthropods
Dung beetles
Dung beetles, particularly the species that roll dung balls, are charismatic species (Scholtz et al, 2009) and they can also perform valuable ecosystem services (Nichols et al, 2008; Beynon et al, 2015). In northern Europe, the predominant species in the coprophagous beetle fauna are endocoprids in the genus Aphodius, which inhabit pats but do not transport faeces away from the pat by burrowing or rolling; less numerous are species of the genus Geotrupes, which are large tunnelling beetles, and other tunnellers and rollers of the genera Copris and Onthophagus (Hanski, 1991). Cow pats are also commonly colonised by predaceous beetle species from the families Hydrophilidae and Staphylinidae. Adult dung beetles have mouthparts that filter out all but the smallest particles (≥20 µm) of dung and their diet is predominantly liquid (Holter and Scholtz, 2007), while the larvae are bulk-feeders and ingest faeces indiscriminately (Holter, 1974).
Dung flies
Adult flies of both pest and non-pest species visit cow pats to feed on surface fluids, to prey on other insects or to mate and lay eggs on the surface (Figure 1), but they are not strictly coprophagous and do not colonise the interior of the pat. Larvae of different species of fly that are present in dung may be coprophagous, but many are carnivorous; in addition hymenopteran parasitoids that prey on dipteran pupae can also be found in dung. Other arthropod groups that occur in dung are the Collembola, or springtails, which are essentially part of the soil fauna, but which can be found in aged dung, and the Acari (mites), which include saprophagous and coprophagous species.
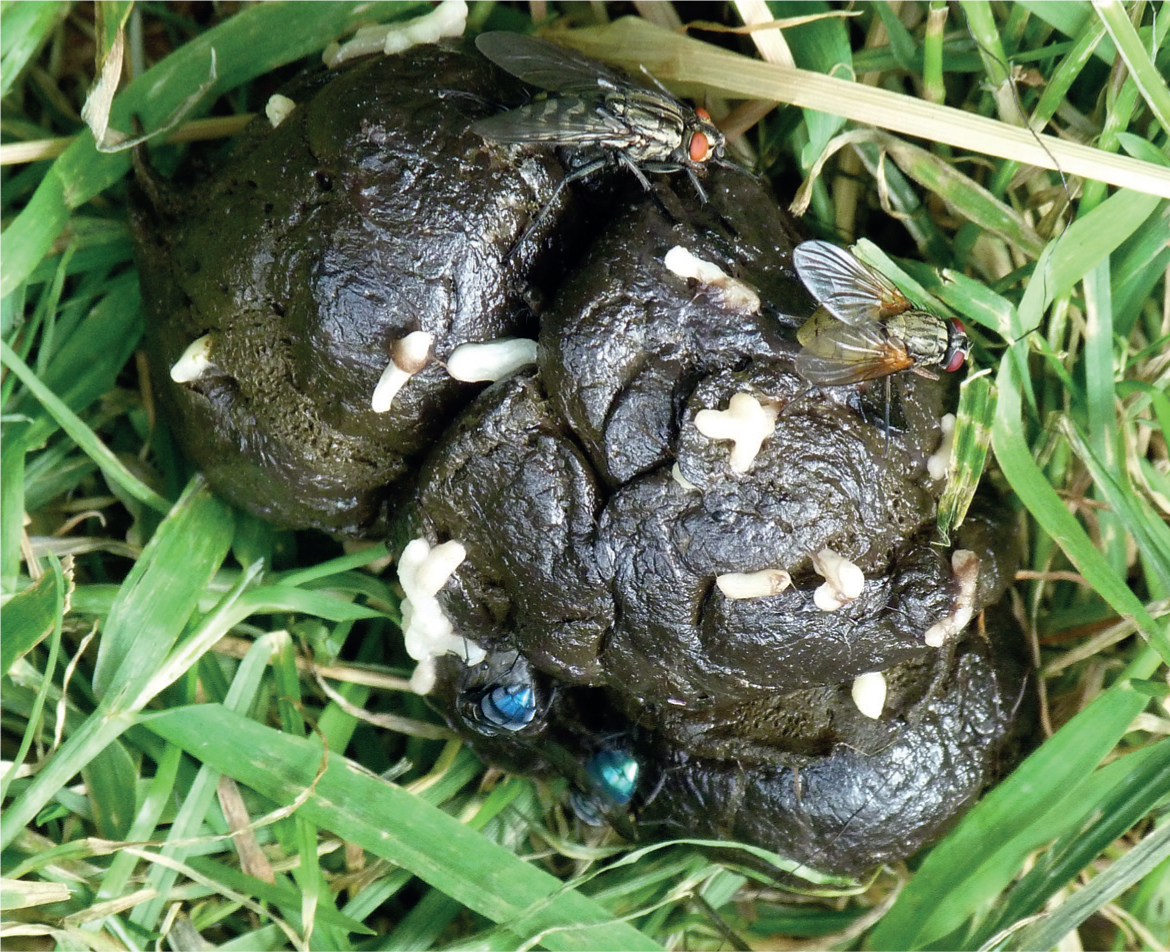
Location, attraction and colonisation of faeces by dung insects
The bovine dung pat is a discrete, variable and ephemeral habitat (Hanski and Cambefort, 1991); its attraction to most adult dung beetle and fly species is highest immediately after deposition and declines over the following days at a rate dependent on factors such as its moisture content (Figure 2). Examples of flies with coprophagous larvae include horn flies, Haematobia irritans, which are haematophagous as adults, feeding on cattle; females lay their eggs in fresh cow dung (Kuramochi, 2000) and have been observed landing on dung as it is falling to the ground when the cow defaecates (Sanders and Dobson, 1969). Peak oviposition is within 2 minutes of faecal deposition and flies favour dung with a moisture content of 84–90% each fly can lay around 10–20 eggs in 3–4 batches under and around the perimeter of the pat (Kuramochi, 2000).
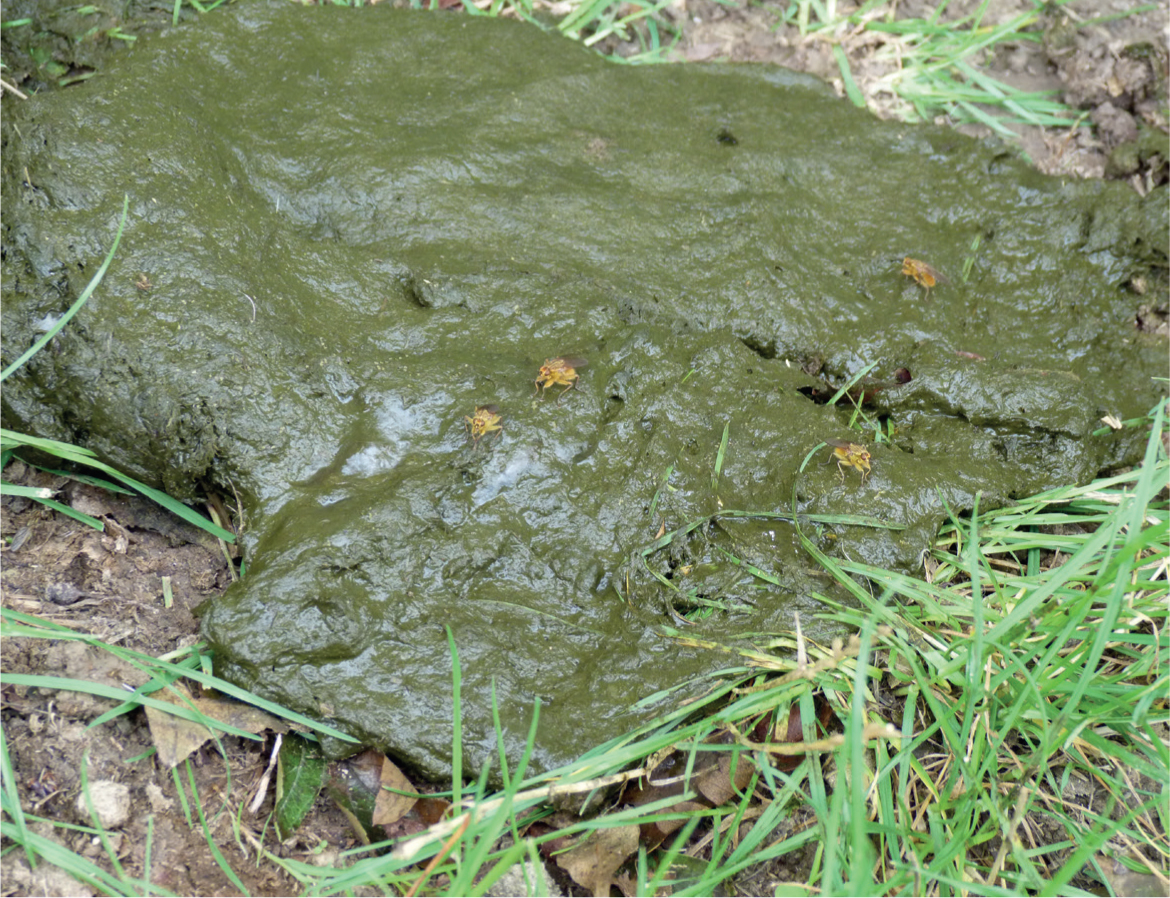
Adult Aphodius dung beetles may live up to 2 months during their breeding season when they visit ~10 different, freshly deposited, pats, staying in each one for around 5 days (Hanski, 1980). Dung is located by olfaction from long distances and by sight when close to the pat. There are two types of olfactory cues for coprophilic insects; these have been subject to most research in dung beetles:
- Cues emanating from volatile constituents of the faeces, which provide insects with information on the location of dung and also its type (herbivore/omnivore/carnivore) (Dormont et al, 2010; Frank et al, 2017,)
- Semiochemicals (pheromones) emanating from insects that have already colonised the dung, providing clues as to the quality of the dung and also the insect communities present, which could represent mating opportunities or competition (Vuts et al, 2014).
These two mechanisms do not operate in isolation and both may be involved in the colonisation of pats by dung beetles (Dormont et al, 2010). Because dung insects can discriminate pats on the basis of smell, aggregation is commonly observed (Wall and Lee, 2010b), so some pats may contain high numbers of insects, while others are ignored.
Earthworms
Earthworms are well known soil invertebrates belonging to the Order Oligochaeta; factors that affect numbers and distribution of earthworms include climate, soil pH, moisture, temperature, organic matter content and agricultural practices (Edwards and Lofty, 1972; Feller et al, 2003). Many species of earthworms are detritivorous and are attracted to substrates rich in organic matter, including animal dung; several species are found in higher densities in soil underlying cow pats than in surrounding areas of pasture (Knight et al, 1992; Bacher et al, 2018). In temperate regions particularly, earthworms play an important role in dung degradation not only through physical disruption of the pat and translocation of the faeces into the soil, but also by digesting the faeces (Hendriksen, 1991a,b).
The dung-pat community
Although a dung pat may seem to be a fairly simple structure with limited biological complexity, in fact it can serve as a habitat for diverse invertebrate fauna, fungi and bacteria, which in turn are integral to food-chains that extend to insectivorous birds and mammals (Jones, 1990; Beintema et al, 1991; Skidmore, 1991). The location, size and composition of dung pats are important factors in the initial colonisation, viability and breeding success of the biota (Finn and Giller, 2000; Holter, 2016, Frank et al, 2017), but the characteristics of dung change over time because of weathering, disturbance and the succession of species with different ecological niches (Lee and Wall, 2006a; Rosenlew and Roslin, 2008; Sladecek et al, 2017). Within the dung, inter- and intra-specific interactions occur, some of which are competitive, some complementary (Holter, 1979a; Wallace and Tyndale-Biscoe, 1983; Hu and Frank, 1996; Finn and Gittings, 2003).
Competitive interactions among the dung fauna can be exploited in actions taken to reduce populations of pest species. One of the reasons behind the programme to introduce exotic dung beetles into Australia was to help control bush flies (Musca vetustissima) and buffalo flies (Haematobia irritans exigua), the larvae of which are coprophagous (Tyndale-Biscoe and Vogt, 1996). The effect of dung beetle activity on helminth transmission is variable (Nichols and Gomez, 2014); dung insects can reduce the risk of nematodosis (Fincher, 1975; Sands et al, 2017), but can also increase the survival and transmission potential of parasitic nematodes (Devaney et al, 1990; Chirico et al, 2003; Nichols and Gomez, 2014).
Dung decomposition
The breakdown of cattle dung is a highly variable process (Barth et al, 1994) and total disappearance of a pat can take anything from a few weeks to over a year (Putman, 1983). The sequence of events and factors that influence the natural rate of degradation include:
- Dehydration
- Initial water content
- Ambient temperature
- Leaching
- Rainfall
- Surface area and thickness of pat
- Fragmentation, aeration and translocation
- Rainfall
- Adult dung beetles
- Earthworms
- Endocoprid insect larvae
- Insectivorous birds amd mammals
- Trampling by livestock
- Digestion and decomposition
- Dung beetle larvae
- Earthworms
- Other soil invertebrates
- Soil-associated fungi and bacteria.
Fragmentation is an important factor in the decomposition of dung and it occurs as the result of heavy rainfall, general weathering and disturbance by grazing animals, foraging birds and wild mammals (Laurence, 1954; Anderson and Merritt, 1977) (Figure 3). Foraging in dung pats varies seasonally, for example, during autumn and winter up to 66% of cattle dung pats were pecked open and scattered by birds, but the effect was less pronounced in spring and summer when only 15–30% were affected (Denholm-Young, 1978) cited in (Putman, 1983). Fragmentation of the dung influences its subsequent rate of decomposition and dung-pat fragments can lose 20–30% more weight in the first 50 days after deposition than intact dung pats, presumably through an increase in the surface/volume ratio, which accelerates dehydration and increases aeration (Denholm-Young, 1978).
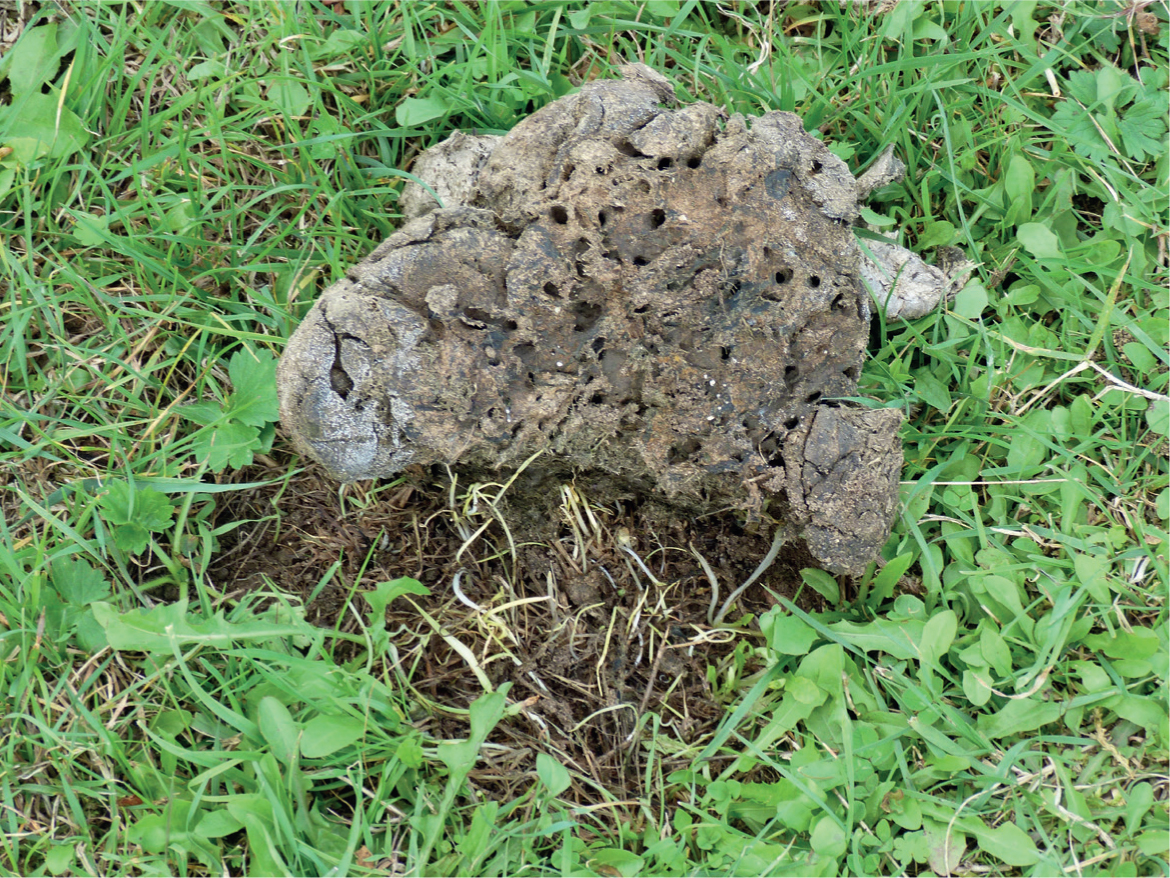
The role of macro-invertebrates in dung decomposition under northern European conditions varies seasonally according to the phenology of the various component species; furthermore their behaviour is strongly influenced in the short-term by environmental temperature and rainfall. Unlike the symbolic dung beetles that roll dung away from the pat, the common beetles in Europe are species of Aphodius, which do not physically remove dung from the pat, but merely burrow through it; nonetheless, they can play an important role by aerating the pat, which can help the development of insect larvae and colonisation by earthworms, which facilitate disintegration of dung. Although Aphodius spp. beetles and their larvae contribute to the disappearance of faeces from pasture (Lee and Wall, 2006b), they commonly assume a secondary role to that of earthworms, which are generally accepted as having the greatest impact on the rate of cow pat degradation in northern Europe (Van Dijk and Bastiman, 1976; Holter, 1979b, Gittings et al, 1994).
Effects of veterinary insecticides on the degradation of bovine dung pats
From the foregoing, it is clear that the decomposition of dung is a multifactorial process in which insects play an important role, but which are responsible for only a small percentage of the overall decomposition process (Holter, 1975; Putman, 1983). Thus, though there are grounds for assuming that insecticidal compounds could delay dung decomposition (Anderson et al, 1984), early studies showed no adverse effects (Miller and Pickens, 1973; Schmidt, 1983), but subsequently significant delays in the disappearance of dung from ivermectin-treated animals were reported (Wall and Strong, 1987). However, in long-term field studies conducted over 2 or 3 years, no effects on dung disintegration and a variety of other agronomic measurements were observed in fields grazed by cattle treated with long-acting ivermectin boluses compared with controls (Wratten et al, 1993; Dimander et al, 2003).
Methodology is important in studies on dung disintegration and techniques do vary among different trials, but there are some key features that should be incorporated, including adequate replication (Wall and Lee, 2010b) and balancing of potentially confounding factors, such as moisture content and composition of the pats, which are known to influence both colonisation by invertebrates and dung degradation (Barth, 1993). Even when conducted under similar protocols, the results of studies on the effects of MLs on dung degradation are equivocal with some showing delays and some not, but the majority do not show significant retardation in decomposition (Tixier et al, 2016).
Reasons for some of the discrepancies between predictions and results of different studies include:
- Limited/no effect of ML faecal residues on adult dung beetles and non-pest flies
- Lack of effect of ML faecal residues on earthworms (Scheffczyk et al, 2016)
- Relatively small role played by insect larvae in the disintegration of dung pats
- Lack of adverse effects of ML residues on bacteria and fungi
- Importance of other factors such as rainfall
- Methodological differences and deficiencies.
Several of these elements are reviewed in the introduction and discussion of a paper that includes aspects of dung removal (Manning et al, 2018). Overall, the speculation that the use of MLs in cattle would increase pasture fouling and have negative agronomic effects does not appear to have been confirmed under typical farming conditions.
Effects of synthetic pyrethroids (SPs) and triclabendazole
It was not until the 1990s that the effect of treatment with SPs on dung insect fauna was first investigated; effects on adult, but not larval beetles (Onthophagus gazella) were measured in this study. Compared with controls, increased mortality (36–61%) was observed over the 21-days following treatment, with the greatest effects seen within the first few days; beetle mortality was somewhat greater following the use of pour-ons compared with sprays (Bianchin et al, 1992). Subsequently, the results of a number of trials on SPs have been published, looking at both dung beetles and dung flies; increased mortality in adult insects is seen, typically over the first week of treatment and other lethal and non-lethal effects on adults and larvae have been noted (Wardhaugh et al, 1998; Krüger et al, 1999; Sommer et al, 2001; Mann et al, 2015).
Adverse effects vary among different SPs and their formulations, thus flumethrin appears to be largely without measureable activity against the dung insect species tested (Krüger et al, 1998, 1999, 1998) and cypermethrin sprays have minimal effects compared with pour-ons (Kryger et al, 2006), particularly if targeted to the lower limbs and under-belly for tsetse control (Vale et al, 2015). In southern Africa, where SPs are used at high frequency in cattle to control ticks and tsetse flies during seasons when dung beetles are also active, mitigation of adverse effects is a high priority (Vale and Grant, 2002; Sands et al, 2018).
The effects of SPs on dung insect populations and dung degradation in the field have received very little attention in the UK and Eire, though they can be also used at high frequency in cattle of all ages to control ticks, nuisance and biting flies during the grazing season and their use is not constrained in organic systems (Sands and Wall, 2018). Recent studies on the island of Islay in the Inner Hebrides, supported by the Royal Society for the Protection of Birds (RSPB), have shown that treatment with deltamethrin reduces the density of insect larvae in cattle dung (Gilbert et al, 2019); in the same study, adverse effects were also observed following treatment with the flukicide triclabendazole either alone or when given simultaneously with deltamethrin. The toxicity of triclabendazole to insects is not well documented, but there is some evidence in the literature (Kılıç et al, 2015).
The importance of scale in assessing potential ecological impact
The limitations of extrapolation from relatively simple, short-term, small-scale experiments to the field has long been recognised and have been described as ‘Paradigms flossed’, which challenges the assumption that ‘it is possible to extrapolate from the results of single-species laboratory tests in containers low in environmental realism to natural complex ecosystems' (Cairns, 1992). This applies to research on dung insect species that have been shown to be highly sensitive to MLs in controlled, small-scale experiments, but whose abundance was not reduced in longer-term field studies (Table 1).
Table 1. Lack of association between ivermectin toxicity in dung insects and their populations in the field following treatment of young cattle during the grazing season to control parasites
Species/guilds | Laboratory/individual pat studies | Field studies |
---|---|---|
Aphodius spp. | Larval mortality (Strong and Wall, 1994) | Dung beetle abundance increased (Webb et al, 2010) |
Scatophaga stercoraria | Larval mortality + sub-lethal effects (Strong and James, 1993) | Yellow dung fly abundance unaffected (Webb et al, 2007) |
Similar patterns have been observed in studies in Africa, where O. gazella, and Euoniticellus intermedius, native species that have also been introduced into Australia, showed high larval mortality when exposed to ivermectin under controlled conditions (Sommer et al, 1993; Krüger and Scholtz, 1997), but the abundance of the species was unaffected following treatment of cattle under typical farming conditions (Krüger and Scholtz, 1998a,b; Kryger et al, 2005).
There are several reasons that might explain the apparent discrepancies between the results of small and large-scale studies in relation to the possible effects of veterinary parasiticides on the non-target, insect dung fauna, among which two stand out: exposure and natural regulatory and adaptive processes among dung insect communities.
Exposure
It is self-evident that adverse effects on insects, individually or collectively, can only occur when susceptible species are exposed to concentrations of residues to which they are sensitive. The coincidence of insects and residues depends on the seasonality of treatment, the proportion of the herd treated, the excretion patterns of the drug following administration and the phenology of susceptible insects. Thus at spatial scales from fields to the farm and beyond and over temporal scales spanning at least one grazing season, the proportion of susceptible dung insects exposed to potentially toxic residues is typically quite small, even with relatively high parasiticide use scenarios within cattle herds (Forbes, 1993).
Natural regulatory and adaptive processes among dung insect communities
When susceptible insects do encounter toxic residues, the outcomes are either sub-lethal effects, which may limit their viability, or death. Mortality over and above the normal rate and lower birth-rates can reduce population sizes unless compensatory mechanisms are activated. An example of a common response in biology is density-dependent reproduction, whereby reproductive success is higher when population density is lower; this has been demonstrated in dung beetles (Holter, 1979a) and dung flies (Lysyk, 1991), and it may provide a mechanism for the recovery of perturbed dung insect communities.
Another means of population recovery is through immigration from adjacent refugia, which could be untreated domestic animals or wildlife, given that many species of dung insects are generalists that feed on dung from different mammalian species and also other substrates such as detritus (Frank et al, 2017). Because dung is an ephemeral and patchy resource, it is essential that dung insect fauna are mobile, and with one or two notable exceptions (Scholtz et al, 2009) all can fly locally over distances of a few kilometres (Byford et al, 1987; Roslin, 2000), but also over longer distances when they colonise unoccupied niches (Fincher et al, 1983; Krafsur and Moon, 1997).
Good examples of the powers of recovery of dung insect populations can be found in studies on the control of horn flies, in which both the adult flies on the bovine host and the larvae in the dung are killed for several weeks after treatment with topical ivermectin, nonetheless, once efficacy declines, adult fly populations quickly return to their pre-treatment levels (Lancaster et al, 1991; Marley et al, 1993).
Large-scale field studies of the environmental impact of veterinary insecticides
The most direct measure of potential environmental impact of veterinary insecticides comes from field trials conducted over several consecutive years using multiple, matched paddocks or farms. Precise replication is almost impossible to achieve at these scales and outcomes can also be confounded by the vagaries of local weather patterns, heterogeneous landscapes and different farming practices, nonetheless they arguably provide the best available evidence for environmental impacts (Wratten and Forbes, 1996; Wall and Beynon, 2012).
An example of such a study is one conducted under the auspices of the RSPB over 2 consecutive years in south-west Scotland, incorporating 14 fields on seven stock farms in which youngstock were treated with either ivermectin or doramectin according to typical farm practice, which comprised two treatments ~8 weeks apart commencing at or soon after turnout in spring. There were no significant differences in either year in the populations of the yellow dung fly, Scatophaga stercoraria, in fields grazed by treated or control cattle (Webb et al, 2007). Over the 2 years of the study, dung beetle populations were significantly higher (9377) in fields in which treated cattle grazed, versus 2483 beetles in fields with untreated cattle (Webb et al, 2010).
There were significant differences in dung beetle (Aphodius spp.) guilds associated with treatment in this study (Webb et al, 2010) and this is characteristic of research elsewhere in which dung beetle abundance may not be reduced in pastures grazed by ML-treated cattle, but the species composition can change (Krüger and Scholtz, 1998a), though these effects vary according to season and prevailing weather conditions (Krüger and Scholtz, 1998b; Sands and Wall, 2018).
Colonisation of faeces from treated animals
The results of preference studies in the literature, based mainly on MLs and dung beetles, are consistently inconsistent, showing attraction, no-effect and repellence (Holter et al, 1993; Floate, 1998), the outcomes varying according to factors that include protocol, compound used, insect species present and time after treatment, but with no discernible, consistent pattern. Though some researchers addressed possible causes of these observations, it is known from their basic chemistry that all the MLs tested are essentially non-volatile, particularly at environmental temperatures; furthermore, they are metabolised to a limited extent in ruminants and the primary metabolites are equally non-volatile (Andrew and Halley, 1996). Hence there is no valid scientific explanation for differences in attraction that can be associated directly with the presence or magnitude of chemical residues. When/if differences in attraction occur, they can only be the result of secondary changes in the volatile compounds known to affect insect behaviour, which could occur following the removal of gastrointestinal nematodes, which can influence faecal components such as the number and diversity of gut bacteria and the water-content of faeces (Jennings et al, 1966; Armour, 1974).
‘Epidemiological’ studies
Associations between dung beetle abundance and farming systems have been studied in the UK and Ireland, focusing on differences between organic and conventional farms. In Ireland, dung beetle abundance and diversity in cattle dung were greater on organic farms compared with conventional lowland farms and farms with rough grazing. The organic farms did not apply artificial fertiliser to the fields, nor did the farmers use MLs, and the absence of these practices may have contributed to the observed differences, however, the authors also noted additional differences in vegetation (hedges and trees) surrounding organic fields and the presence of other livestock species, which can enhance beetle diversity (Hutton and Giller, 2003). In a similar study in the UK, there were no differences in dung beetle abundance between farms that used SPs, MLs or no insecticides over the summer, but differences in species composition were evident in late summer, notably in the paracoprid species (dung burying beetles), which were under-represented in farms that used MLs (Sands and Wall, 2018). However, similar trends in the abundance of dung insects between organic and conventional farms have also been observed in the absence of any parasiticide treatments (Figure 4) (Geiger et al, 2010), suggesting that organic pastures and systems can be intrinsically more favourable for the insect fauna of cow pats, irrespective of the use of veterinary treatments.
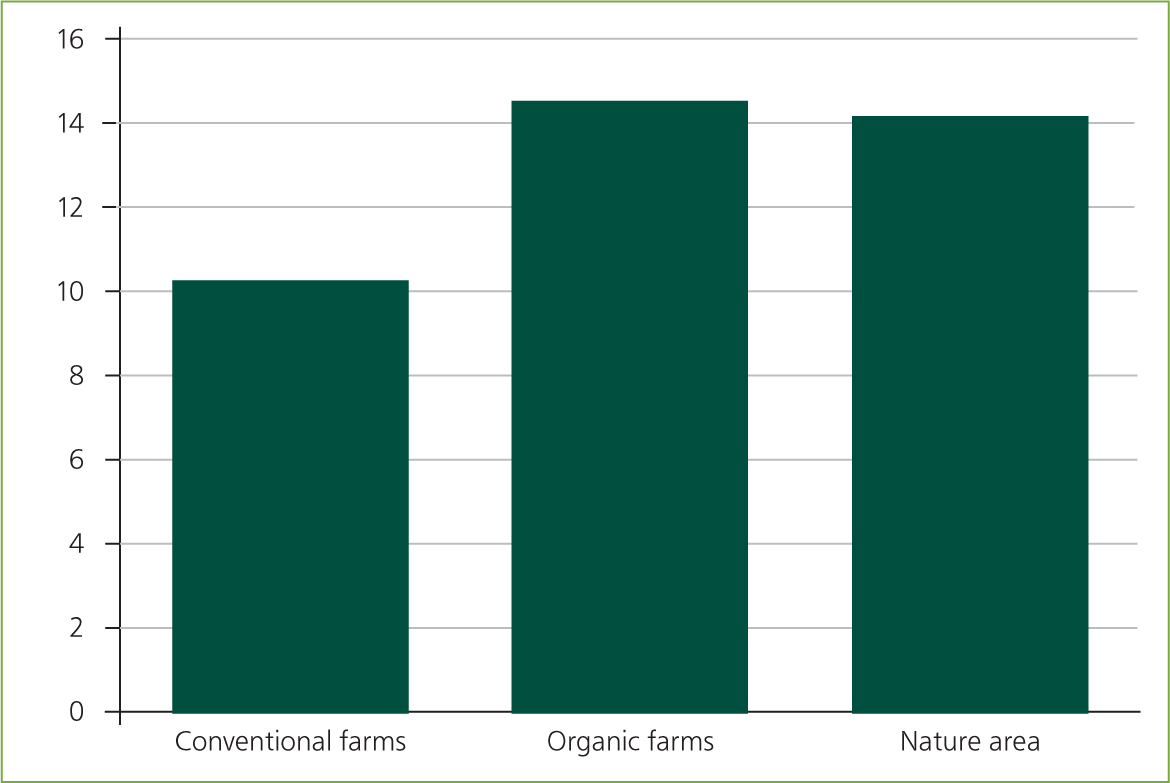
Concerns have been raised that the use of MLs could affect populations of the red-billed chough (Pyrrhocorax pyrrhocorax) by restricting the food supply of young birds (McCracken, 1993), which feed on dung invertebrates at certain times of year (McCracken and Foster, 1994). However, a study on parasite control practices at different locations in the UK where choughs breed found that MLs were used similarly in all areas, but on Islay, the only site where chough populations are declining, significantly higher use rates of pyrethroids and triclabendazole occurred compared with other sites (Jonsson et al, 2020). Products containing SPs and triclabendazole are commonly used to treat and control fasciolosis and ticks on the island, whereas these parasites are not subject to routine treatment at the other sites.
Mitigation practices
Irrespective of how the environmental impacts of veterinary medicines are judged, limiting exposure of both target and non-target species is a consistent objective within the overall context of responsible use, which is also promulgated for the management of parasiticide resistance (Sherratt, 1998; Cooke et al, 2017). Refugia of non-target insects are always present, but their magnitude in both space and time varies according to a multitude of factors, but refugia enhancement should be an important objective for live-stock farmers and targeted group treatment or targeted selective (individual) treatment (TST) should be standard practice (Forbes, 2019; Greer et al, 2020). Mitigation practices are described below.
Refugia recognition and enhancement
- Dung deposited before treatment, or following treatment once toxic residues have disappeared
- Dung from untreated cattle within the group and elsewhere on the farm
- Dung from other (untreated) livestock and wildlife in the vicinity.
Asynchrony of treatments and insect activity
Some parasiticide treatments are very low risk as they do not coincide with periods when insects are active, for example:
- If animals are treated at housing, not only is fresh dung not deposited on pasture, but the manure and slurry is stored and spread the following year, by which time insecticide residues will have been diluted and degraded
- If animals are out-wintered and treated, there is limited dung insect activity on pasture when temperatures are <10°C
- Dung insects may only be active for some months over the grazing season, e.g. the yellow dung-fly, Scatophaga stercoraria is mainly on the wing in spring and autumn; some dung beetle species are only active at certain times, for example in autumn (Gittings and Giller, 1997).
Product choice
The relative toxicity of different parasiticides can only really be assessed under controlled conditions against a limited array of insect species and such studies do not accurately mimic what happens in the field. Nonetheless, using such approaches, MLs can be compared and ranked, though the results are not consistent (Floate et al, 2001). In general, among the MLs, moxidectin is a less potent insecticide than the avermectins (Vercruysse and Rew, 2002) and this is reflected in its effects on non-target as well as target insects, however, its faecal residues have been shown to have detrimental effects on several dung beetle and fly species, including those of ecological and economic importance (Floate et al, 2001; Steel and Wardhaugh, 2002; Hempel et al, 2006; Manning et al, 2018). Similarly, the SPs differ in their toxicity to non-target insects under controlled conditions, with some having fewer adverse effects in the species tested than others, but to apply the moniker of ‘dung beetle friendly’ to any veterinary product with insecticidal activity seems to be misleading, inappropriate and unjustified by the available scientific evidence.
Among the anthelmintics, benzimidazoles and levamisole generally appear to lack effect on non-target insects. Likewise, apart from triclabendazole, there is little evidence that flukicide residues have adverse effects on dung fauna, though some have therapeutic activity against haematophagous, parasitic insects (Swan, 1999).
Dung insect (beetle) conservation
Maintaining and restoring biodiversity is an important driver for conservationists, the public and politicians; for insects in general, there is evidence of recent population declines (Harvey et al, 2020), even in nature reserves (Hallmann et al, 2017), though there have been some challenges to these observations regarding the methodology (Saunders, 2019; Saunders et al, 2020).
The natural abundance of dung insects in their preferred habitats is a function of increases through reproduction and immigration and decreases through mortality and emigration, but in agricultural settings there may be additional threats from human activity. In a recent report: ‘Review of the status of the beetles of Great Britain: the stag beetles, dor beetles, dung beetles, chafers and their allies’ (Lane and Mann, 2016), the following practices were listed as potential threats to the dung insect fauna:
- Reduction of traditional land management and loss of habitats
- Development of (rural) land for houses, amenities etc
- Loss of permanent pasture
- Pasture improvement
- Harrowing, ploughing, re-seeding, fertilising, changes in dung composition
- Changes in livestock grazing practices
- Cessation of livestock grazing (and hence dung supply)
- Prophylactic use of parasiticides.
This report does not contain any observations on the current populations of common dung beetles, but does provide evidence for the decline or local extinction of some 18 rare species, several of which are associated with the dung of sheep, cattle, horses and rabbits (Lane and Mann, 2016). Records for many of these species are from specialised habitats such as sand dunes, coastal grassland and chalk downland and in several cases the declines or disappearance preceded the introduction of the MLs in the late 1980s.
Conclusions
Collectively, the scientific literature on potential environmental impacts resulting from the use of veterinary parasiticides, examples of which are cited here, indicates that the effects at farm and landscape scales over ecologically relevant time periods are less than had been predicted from short-term, small-scale studies. An explanation may be that the adaptation and resilience shown by dung insects in the face of perturbations has been underestimated; in controlled field and mesocosm studies, it has been shown that biodiverse dung beetle communities can compensate for ivermectin-induced perturbations (Beynon et al, 2012; Manning et al, 2017). Other considerations are that treatment frequency and intensity have been over-estimated and the role of refugia has been underestimated. This subject remains quite controversial, as reflected in the disparate views held by members of the scientific community; this article provides an interpretation of the scientific evidence base as it relates to dung fauna and the potential impact of parasiticides. Objective, quantitative environmental impact assessments, based on the available science, are germane to appropriate management, mitigation and conservation practices for the dung fauna while giving due consideration to the need to control parasites, keeping livestock healthy and productive (Pullin and Knight, 2003).
KEY POINTS
- All veterinary products must undergo environmental risk assessments prior to licensing.
- Among veterinary treatments for farm animals, those with insecticidal activity have the potential to affect non-target invertebrates.
- Under controlled, small-scale studies, several veterinary parasiticides have adverse effects on some insect species in the dung fauna.
- Faecal residues of veterinary insecticides have the potential to adversely affect dung insect populations and their ecological roles.
- Although adverse effects have been observed in some large-scale farm studies, generally the impact is less than would have been predicted from toxicity tests.
- Refugia-based use of veterinary parasiticides can reduce and mitigate the potential for adverse environmental impact.