Pleuropneumonia is a highly contagious respiratory disease of swine which can be caused by Actinobacillus pleuropneumoniae infecting the lungs and resulting in fibrinous pleurisy and pneumonia (Gottschalk and Broes, 2019). The infection can manifest as acute, subacute and subclinical, which can quite often develop into chronic disease. The disease can have a large economic impact because of increased mortality as a result of acute deaths, increased feed conversion ratio, decreased growth rate and increased medication costs for treatment of affected animals (Sassu et al, 2018), as well as increasing susceptibility of the animal to secondary infections (Dubreuil et al, 2000). The disease can be difficult to control because of subclinically infected animals or those recovered from clinical cases acting as carrier animals on the farm, continuing to provide a source of infection to other animals. Therefore, ensuring effective control of the disease is very important for producers. Implementation of a vaccination programme is one of the main methods used in a control plan for pleuropneumonia, and has been shown to be effective in reducing clinical cases and also the impact of the disease in terms of lung lesions and losses in productivity (Cvjetkovic et al, 2018).
The pathogen
A. pleuropneumoniae is a Gram-negative encapsulated coccobacillus bacterium which produces fine fimbriae, capsules and secretes toxins. The bacterium is classified based on its requirement for nicotinamide adenine dinucleotide (NAD) for growth, with bio-type 1 being NAD dependent and biotype 2 being NAD independent (Gottschalk and Broes, 2019). There are numerous different strains of A. pleuropneumoniae and the virulence differs greatly between these, with highly virulent strains often resulting in acute deaths, therefore causing large increases in mortality. However, strains of a particular serotype may be highly virulent in one region but not in others, showing the unpredictability of the disease. In total there are 18 A. pleuropneumoniae serotypes identified to date (Bossé et al, 2017a, 2018). The prevalence of serotypes differs by country, for example, in England and Wales samples taken from 2008–2014 showed serotype 8 to be dominant (Li et al, 2016). Farms can be infected with one or more serotypes and the virulence of the strains within these can vary.
Serotypes are characterised by differences in the capsular polysaccharides (Dubreuil et al, 2000) which are associated with particular lipopolysaccharides. In some cases, different capsular polysaccharides are associated with the same cell wall lipopolysac-charides which explains the cross reactivity to lipopolysaccharide antigen observed across multiple serotypes, for example between serotypes 1, 9 and 11 (Gottschalk and Broes, 2019).
Together with capsular polysaccharides and lipopolysaccharides, the extent of the virulence of the serovars is mainly determined by the four different proteinaceous cytotoxins of A. pleuropneumoniae, ApxI, ApxII, ApxIII, and ApxIV (Table 1), which belong to the pore-forming repeat-in-toxin family (Frey, 1995).
Table 1. Toxin production from different Actinobacillus pleuropneumoniae serotypes
Apx toxin | A. pleuropneumoniae serotype | |||||||||||||||||
---|---|---|---|---|---|---|---|---|---|---|---|---|---|---|---|---|---|---|
1 | 2 | 3 | 4 | 5 | 6 | 7 | 8 | 9 | 10 | 11 | 12 | 13 | 14 | 15 | 16 | 17 | 18 | |
I | x | x | x | x | x | x | ||||||||||||
II | x | x | x | x | x | x | x | x | x | x | x | x | x | x | x | x | ||
III | x | x | x | x | x | x | ||||||||||||
IV | x | x | x | x | x | x | x | x | x | x | x | x | x | x | x | x | x | x |
Epidemiology
In animals of all ages, A. pleuropneumoniae will be located at the tonsils when the animal is initially infected. While here, the bacteria are inactive and not involved with the immune system. These pigs are passive carriers able to infect others but not identifiable in serology, hence posing high risk in transmission of A. pleuropneumoniae. A combination of factors including lack of immunity, general stress and/or other respiratory disease challenge can result in the bacteria moving to the lungs. Manifestations can be peracute, acute, subacute or subclinical producing pneumonic and pleural pathological changes as well as meeting the immune system; subsequently being identifiable via serology or isolation from affected lung tissue. All of these manifestations are likely to develop into chronic stages identifiable for months, even at the time of slaughter. At all stages of infection, the pig is considered capable of transmitting disease to other animals, via the tonsils and both the upper or lower respiratory tract, including micro-abscesses in lung tissue. This explains why pleuropneumonia is a difficult disease to control on farm and in some circumstances, producers may choose to depopulate the unit in an attempt to eliminate the pathogen.
Outside the host, A. pleuropneumoniae is not particularly persistent in warm, dry environments, but can however survive for weeks when covered with biofilm, organic matter or mucus (Gottschalk and Broes, 2019), therefore reinforcing the importance of good hygiene and disinfection protocols on the farm. Effective removal of organic matter via high-pressure washing, biofilm via soap immersion of at least 10 minutes plus subsequent high-pressure washing, and use of a disinfectant on the dried surfaces can remove the bacteria from buildings and equipment in the pens such as drinkers and feeders (Gottschalk and Broes, 2019).
Transmission between herds can occur via introduction of new animals, most likely breeding stock, which are carriers of A. pleuropneumoniae. Therefore, there is benefit in running a closed herd with high standards of both internal and external biosecurity. Transmission within the herd occurs most commonly via nose to nose contact between animals or by droplet transfer over short distances (Gottschalk and Broes, 2019). The likelihood of large outbreaks on the farm increases if animals are mixed, resulting in transmission between groups of pigs. It is important to note the role of sows, particularly young sows with no or low immunity to the relevant A. pleuropneumoniae serotype, actively excreting A. pleuropneumoniae which can be transmitted to piglets, with the risk of transmission increasing as bacterial excretion increases. Although, some piglets may be protected by their maternally derived antibodies, which in the case of A. pleuropneumoniae have been shown to be present anywhere from 2 weeks to 2 months, this will vary from farm to farm (Vigre et al, 2003).
Pathogenesis
There are three stages of pathogenesis for A. pleuropneumoniae: colonisation; evasion of host defences; and damage to host tissues (Bossé et al, 2002). Following exposure of the animal to A. pleuropneumoniae, the bacteria colonises the surface epithelial cells of the respiratory tract causing them to vacuolate and desquamate (Gottschalk and Broes, 2019). The lower respiratory tract is the preferred site for A. pleuropneumoniae binding, with the bacterium binding to mucus, proteins and ciliated epithelial cells in the bronchi and alveoli (Dom et al, 1992; Chiers et al, 2010). The presence of fimbriae has been shown to promote adherence of bacteria to cells, and therefore the colonisation of the respiratory tract in the case of A. pleuropneumoniae will be assisted (Zhang et al, 2000). Lipopolysaccharide has also been reported to play an important role in achieving optimal adhesion of A. pleuropneumoniae to respiratory tract cells (Ramjeet et al, 2005). Biofilm formation is prevalent in A. pleuropneumoniae (Kaplan and Mulks, 2005), in which surface-associated colonies of bacteria form, embedded in an extracellular substance that enables attachment to the underlying surface (Chiers et al, 2010). Biofilms protect an organism from environmental challenges such as components of the cell's immune system, antimicrobials and disinfectants and can therefore increase persistence of A. pleuropneumoniae in a herd; in a recent study, 71% of A. pleuropneumoniae isolates were able to form biofilms and this was considered positively correlated to virulence (Aper et al, 2020).
The secretion of Apx toxins results in lysis of alveolar cells, endothelial cells, red blood cells and lymphocytes including neutrophils and basophils (Chiers et al, 2010). Lipopolysaccharides in the cell wall and A. pleuropneumoniae toxins attract macrophages and neutrophils and stimulates macrophages to secrete inflammatory cytokines. Release of oxygen metabolites and lysosomal enzymes results in damage to lung tissues (Bossé et al, 2002). The lipopolysaccharide has this effect because it attracts macrophages and neutrophils and stimulates macrophages to secrete inflammatory cytokines. Release of oxygen metabolites and lysosomal enzymes results in damage to lung tissues (Gottschalk and Broes, 2019).
Clinical signs and pathology
Manifestations and clinical signs vary based on the age of the animal, their immune status and the environmental conditions. Disease can be peracute, acute, subacute, subclinical or chronic and clinical signs differ between these. In peracute cases, clinical signs include high fever, anorexia and apathy. Affected animals may lie on the ground with obvious respiratory signs including mouth breathing and foamy blood-tinged nasal discharge which is usually present shortly before death. Acute cases share some clinical signs including fever and severe respiratory signs including coughing and laboured breathing. The animals may also be reluctant to feed or drink and be generally depressed. The severity and progression of clinical signs is related to the lung lesions present. The subacute and subclinical manifestations are ‘further down the road’ in clinical severity where there are no apparent clinical signs in pigs, which have subsequent positive serology, and in most cases have clearly distinguishable pathological changes. The chronic form of disease occurs following disappearance of any of the clinical manifestations, where treatment or control measures do not effectively prevent disease occurrence. Fewer severe clinical signs are present, with no fever and an intermittent cough. Lethargy and reduced appetite and therefore growth rate may also be observed (Sassu et al, 2018; Gottschalk and Broes, 2019).
The majority of the pathological changes that occur in pleuropneumonia cases are as a result of the Apx toxins and the effects they exert directly on host cells but also indirectly via stimulation of cytokine release from phagocytes (Bossé et al, 2002). Gross lesions are located in the respiratory system including pneumonia and fibrinous pleurisy (Figure 1). In some cases, there are haemorrhagic strands around the necrotic lesion under the pleura (Dubreuil et al, 2000). In acute cases there is dark consolidation, interlobular oedema, and mild to severe fibrinous pleuritis (Figure 2). Chronically infected pigs typically have pleural adhesions, and abscesses may be found in the lung. The lung lesions in uncomplicated infections may resolve completely in only a few weeks, and only pleural adhesions might be seen at slaughter (Marsteller and Fenwick, 1999). A high level of dorsocaudal pleuritis at slaughter suggests previous A. pleuropneumoniae infection (Merialdi et al, 2012).
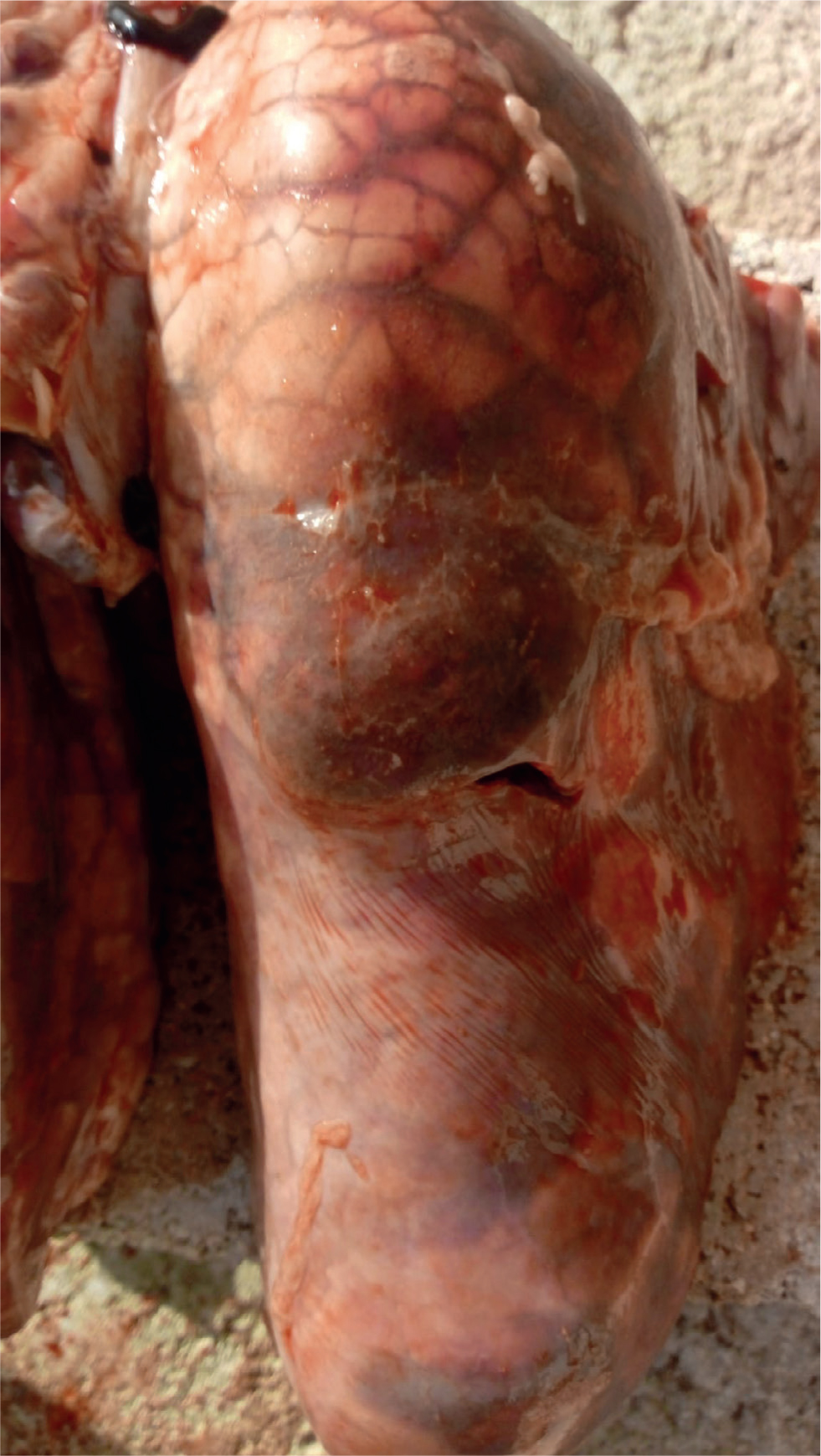
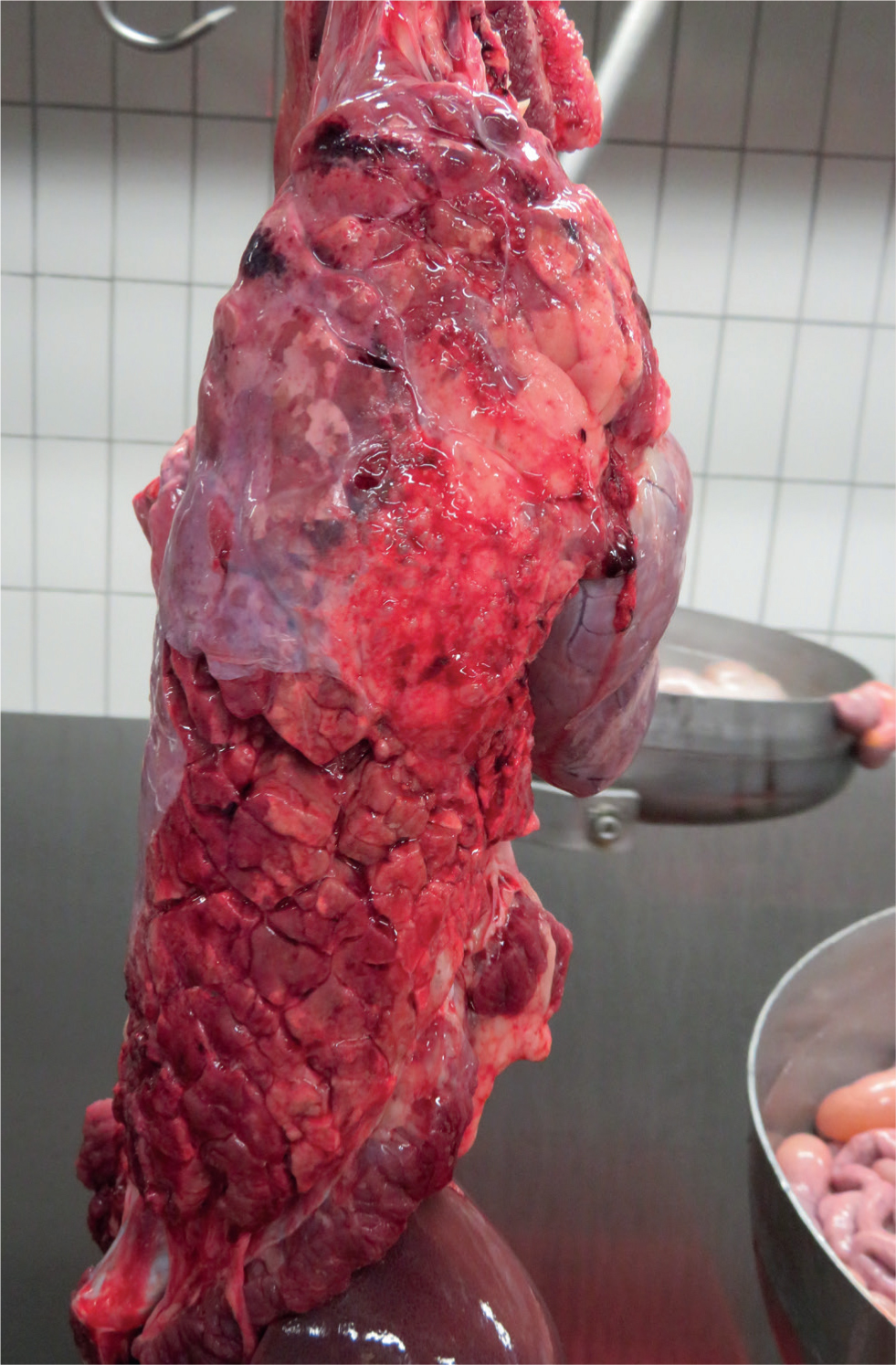
Interactions with other diseases
Certain A. pleuropneumoniae strains can also act as secondary pathogens in combination with other viral and bacterial pathogens, contributing to the development of porcine respiratory disease complex (PRDC) (Gottschalk and Broes, 2019). The importance of the bacterial and viral co-infections with respect to PRDC is significant because these can exacerbate the current respiratory syndromes. The main pathogens involved in PRDC includes porcine circovirus (PCV2), porcine reproductive and respiratory syndrome (PRRS) virus, swine influenza virus (SIV), Mycoplasma hyopneumoniae and A. pleuropneumoniae.
Pigs first infected with M. hyopneumoniae and later with A. pleuropneumoniae present with very severe clinical signs and lung lesions that correspond to the pathogenicity of the two bacterial strains combined (Marois et al, 2009). In the case of influenza, a co-infection between this virus and A. pleuropneumoniae potentiates the severity of lung lesions and enhances viral replication in the lung and the nasal shedding. This replication contributes to a more severe clinical situation (Pomorska-Mól et al, 2017). The mechanism of interaction between A. pleuropneumoniae and PRRS virus still needs to be clarified (Sassu et al, 2018).
Diagnosis
The accurate diagnosis of A. pleuropneumoniae disease is essential to ensure that the most optimal control plan is implemented on the farm. For a correct diagnosis several factors should be considered, such as magnitude and individual variation in maternal A. pleuropneumoniae transfer with focus on young mothers, magnitude and individual variation in maternal-derived immunity, the time taken for infection to enter lung tissue and the need to act fast in cases of acute outbreaks.
As most farms are infected with several A. pleuropneumoniae serotypes, it is very important to correctly diagnose A. pleuropneumoniae. To monitor potentially new serotypes entering the farm, the identification of the A. pleuropneumoniae serotype clinically active is important. The isolation of the serotype involved in the clinical case/outbreak is best achieved by taking lung swabs or sending the lung to the lab after a post-mortem examination in cases of acute outbreaks. The samples should be obtained from untreated animals that present typical acute pleuropneumonic lesions; culture of the pathogen is usually unrewarding in chronic cases.
Deep tonsillar scraping is a practically complicated procedure to get right. However, it holds the best possibility of revealing other (all) serotypes present on the farm, and is the only way to diagnose silent carriers. The fact that tonsillar located A. pleuropneumoniae bacteria are usually embedded in biofilm and at a low-level biological state does not make the full, correct serotype profile easy to determine (Hoeltig et al, 2018). Polymerase chain reaction (PCR) techniques are useful and reliable tools to differentiate unequivocally A. pleuropneumoniae serotypes if needed (Bosse et al, 2014, 2018).
Serological tests will be limited to reveal presence of a specific A. pleuropneumoniae serotype due to cross reaction within serogroups (Gottschalk and Lacouture, 2014). Also, several tests are required to be ensure they are covering the full serogroup range. Currently, only serotypes 1 to 15 are covered, leaving out 16 to 18 and non-typable serotypes. Therefore, serological tests to determine the serotype of the A. pleuropneumoniae present may be a potentially expensive strategy.
To determine whether or not A. pleuropneumoniae is present on farm, the enzyme-linked immunosorbent assay (ELISA) based on ApxIV antibody detection is a very helpful tool, as all the serotypes produce this toxin and animals infected with any of the serotypes can be detected. However, sensitivity can be low, meaning the positive test result has more decision power than the negative (Schaller et al, 2001). This technique is also used to evaluate the dynamics of infection through seroprofiles, particularly the seroprofile evaluating the regression of maternal immunity and determining the time point of increase in antibodies as a result of pulmonary infection (Figure 3). The period between the week before weaning and 12–13 weeks of age are usually covered cross-sectionally: 10 samples should be taken for each age group in 2 to 3 week intervals. The same samples are evaluated for PRRS virus, as avoiding PRRS virus circulation at the time of A. pleuropneumoniae vaccination is crucial because of possible interaction. With this information the best time window can be estimated: vaccination should occur at the time where colostral A. pleuropneumoniae antibodies are the lowest possible, while still leaving 3 week intervals for the two subsequent vaccinations, plus 1 to 2 weeks from last vaccination to pulmonary infection.
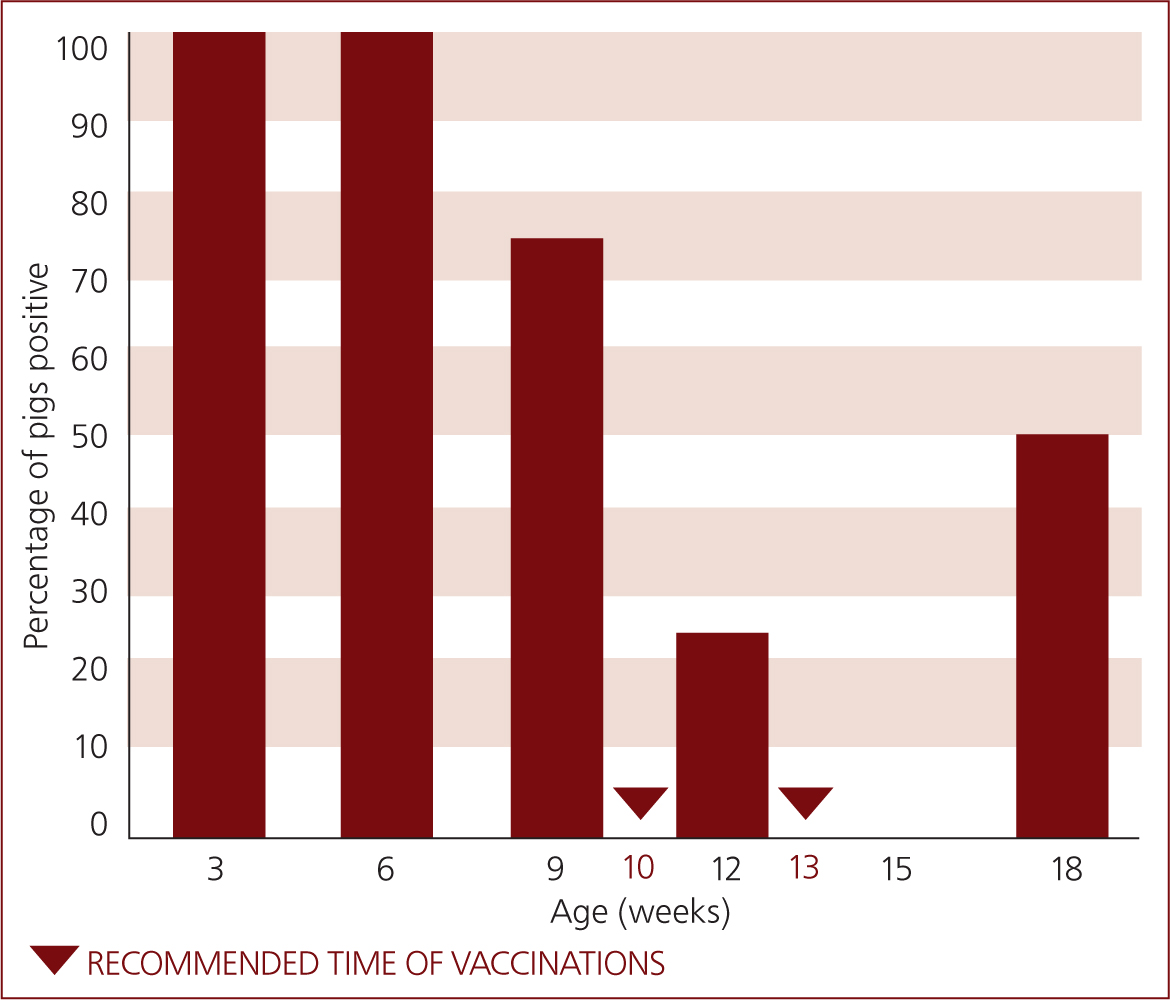
Control and treatment
Various approaches can be taken in controlling pleuropneumonia on farm and the measures taken should be based on assessment of each individual farm's clinical situation. Good husbandry and biosecurity are imperative in controlling disease on farm and can be accompanied by vaccination (Sassu et al, 2018). Use of antibiotics to control outbreaks is effective in reducing the clinical severity of the outbreak through the farm (Papatsiros et al, 2019). However, high resistance rates have been reported to some medicines that are used to treat pleuropneumonia, with an increase in antimicrobial resistance found for the majority of drugs assessed (Vanni et al, 2012). More recent studies support this, showing resistance in particular strains (Bossé et al, 2015), and a study in the UK reported only 33% of pleuropneumonia isolates analysed to be negative for resistance genes (Bossé et al, 2017b). Therefore, other methods for controlling pleuropneumonia on farm are preferable, such as vaccination.
There are different types of vaccines available for vaccination against A. pleuropneumoniae. The types can be split into:
- Bacterins — serotype specific protection only with no protection against the most virulent agents in A. pleuropneumoniae ApxI, II and III,
- Subunit or toxoid vaccines — expressing the potent ApxI, II and III, but not lipopolysaccharides and several other immunological competent cell-wall antigens
- Bacterins expressing Apx I, II and III — including all known protective antigens and serotype-independent protection. The most advanced A. pleuropneumoniae protective concept of today.
The aim of vaccination is to prevent outbreaks, reduce clinical signs if they occur, and most importantly to the farmer, prevent losses in mortality, feed conversion ratio and growth, the latter affecting both pen efficiency and heterogeneity at the time of slaughter. However, it is important to remember that vaccines do not prevent infection. To achieve optimal timing and effectiveness of vaccination of the pigs, emphasis should be placed on ensuring the cross-sectional seroprofiling is completed and avoidance of simultaneous PRRS virus circulation as described above. Focusing on optimising investments on the farm will increase the chance of maximal return on investment.
The effectiveness of a vaccination programme for A. pleuropneumoniae can be evaluated based on a reduction in pleuritis reported at slaughter and a reduction in mortality and use of antimicrobials to control the disease on farm (Cvjetkovic et al, 2018). A study comparing five registered commercial A. pleuropneumoniae vaccines in Europe reported on mortality and average lung lesion score of batches sent to slaughter. Coglapix® (Ceva Animal Health Ltd) as demonstrated in this controlled experimental challenge study, had the highest efficacy in preventing the mortality and development of lung lesions as a result of infections with A. pleuropneumoniae serotype 2 (Kiss et al, 2017). Similar results were found in a study in the UK on a 350-sow unit vaccinating with Coglapix®, with a reduction in dorsocaudal pleurisy from 64% prior to vaccination to 21% in vaccinated animals (Velazquez and Gale, 2019). A study over 2 years on a 1700 sow herd in Spain comparing animals vaccinated with Coglapix® versus unvaccinated animals reported an improvement in feed conversion ratio from 2.61 to 2.49 and improvement in average daily weight gain from 640 to 660 g/day in Coglapix® and nonvaccinated groups respectively (Cárceles et al, 2019). These studies demonstrate the range of benefits in controlling pleuropneumona with vaccination and the economic benefit of a successful vaccination programme.
Conclusion
Porcine pleuropneumonia is a contagious respiratory disease that is of large economic importance for pig producers, quite often exceeding expectations as a result of subacute and subclinical losses on efficacy. Therefore, control strategies including strict biosecurity and hygiene measures and vaccination are imperative in combatting the disease on farm. Vaccination has been shown to be effective in reducing clinical signs of disease and reducing the prevalence of lung lesions at slaughter. Performance benefits of vaccination have also been shown, therefore, reducing the economic impact. Vaccination has also proved superior to antimicrobial strategies alone, hence contributing to a reduction in their general use, as well as their frequent use to treat clinical outbreaks.
KEY POINTS
- Actinobacillus pleuropneumoniae is a respiratory pathogen which can cause disease that can result in losses in productivity on farm.
- Virulence of the pathogen varies between serotypes and location.
- Interactions with other pathogens can also occur and contribute to porcine respiratory disease complex (PRDC).
- Identification of serotypes present on the farm is important as well as seroprofiling to determine levels of maternally derived antibodies present in pigs.
- Implementation of a vaccination programme has been shown to be effective in controlling the disease including reduction in clinical severity and reduced losses.